ACTTEL RTSX72SU
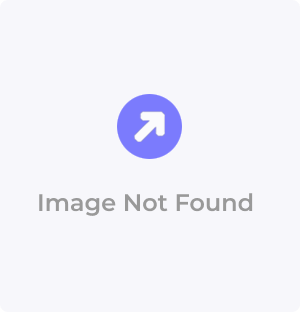
Description
Actel RTSX72SU RadTolerant FPGA designed for enhanced radiation performance
Part Number
RTSX72SU
Price
Request Quote
Manufacturer
ACTTEL
Lead Time
Request Quote
Category
PRODUCTS - R
Specifications
CCGA
624
Clocks
3
Combinatorial Cells
4,024
CQFP
208,256
Logic Modules
6,036
Maximum Flip-Flops
4,024
Maximum User I/Os
360
Quadrant Clocks
4
SEU-Hardened Register Cells
2,012
Speed Grades
Std.,-1
System Gates
108,000
Temperature Grades
B,E,
Typical Gates
72,000
Features
- 100% circuit resource utilization with 100% pin locking
- 230 MHz system performance (310 MHz internal)
- 3.3 V and 5.0 V mixed voltage
- 32,000 to 72,000 ASIC gates (48,000 to 108,000 system gates)
- 5 V input tolerance and 5 V drive strength
- Configurable I/O support for 3.3 V/5 V PCI, LVTTL, TTL, and CMOS
- Hermetically-sealed packages for space applications (CQFP, CCGA/LGA, CCLG)
- Highly reliable, nonvolatile antifuse technology
- JTAG boundary scan testing in compliance with IEEE Standard 1149.1 — dedicated JTAG Reset (TRST) Pin
- Low-Cost prototyping option deterministic, user-controllable timing
- Secure programming technology prevents reverse engineering and design theft
- Unique in-system diagnostic and verification capability with Silicon Explorer II
- Up to 360 user-programmable I/Os
- Very low power consumption (Up to 68 mW at Standby)
Datasheet
Extracted Text
Revision 9 RTSX-SU Radiation-Tolerant FPGAs (UMC) Designed for Space Specifications • SEU-Hardened Registers Eliminate the Need to Implement • 0.25 µm Metal-to-Metal Antifuse Process (UMC) Triple-Module Redundancy (TMR) • 48,000 to 108,000 Available System Gates – Immune to Single Event Upsets (SEU) to LET > 40 MeV- • Up to 2,012 SEU-Hardened Flip-Flops th 2 cm /mg, • Up to 360 User-Programmable I/O Pins –10 – SEU Rate < 10 Upset/Bit-Day in Worst-Case Geosynchronous Orbit Features • Up to 100 krad (Si) Total Ionizing Dose (TID) – Parametric Performance Supported with Lot-Specific Test • Very Low Power Consumption (Up to 68 mW at Standby) Data • 3.3 V and 5 V Mixed Voltage • Single-Event Latch-Up (SEL) Immunity to LET >104 • Configurable I/O Support for 3.3 V/5 V PCI, LVTTL, TTL, and TH MeVcm2/mg CMOS • TM1019.5 Test Data Available – 5 V Input Tolerance and 5 V Drive Strength • QML Certified Devices – Slow Slew Rate Option – Configurable Weak Resistor Pull-Up/Down for Tristated Outputs at Power-Up High Performance – Hot-Swap Compliant with Cold-Sparing Support • 230 MHz System Performance • Secure Programming Technology Designed to Protect Against • 310 MHz Internal Performance Reverse Engineering and Design Theft • 9.5 ns Input Clock to Output Pad • 100% Circuit Resource Utilization with 100% Pin Locking • Unique In-System Diagnostic and Verification Capability with Silicon Explorer II Processing Flows • Deterministic, User-Controllable Timing • B-Flow – MIL-STD-883B • JTAG Boundary Scan Testing in Compliance with IEEE • E-Flow – Extended Flow Standard 1149.1 – Dedicated JTAG Reset (TRST) Pin • EV-Flow – Class V Equivalent Flow Processing Consistent with MIL-PRF 38535 Prototyping Options • Commercial SX-A Devices for Functional Verification • RTSX-SU PROTO Devices with Same Functional and Timing Characteristics as Flight Unit in a Non-Hermetic Package Table 1 • RTSX-SU Product Profile Device RTSX32SU RTSX72SU Capacity Typical Gates 32,000 72,000 System Gates 48,000 108,000 Logic Modules 2,880 6,036 Combinatorial Cells 1,800 4,024 SEU-Hardened Register Cells (Dedicated Flip-Flops) 1,080 2,012 Maximum Flip-Flops 1,980 4,024 Maximum User I/Os 227 360 Clocks 33 Quadrant Clocks 04 Speed Grades Std., –1 Std., –1 Package (by pin count) CQ 84, 208, 256 208, 256 CG 624 CC 256 March 2012 i © 2012 Microsemi Corporation RTSX-SU Radiation-Tolerant FPGAs (UMC) Ordering Information RTSX72SU 1 CQ B Application (T emperature Range) B= MIL-STD-883 Class B E= E-Flow (Space Level Flow) = Military Temperature M EV= Class V Equivalent Flow Processing Consistent with MIL-PRF-38535 = Prototyping Flow PROTO Package Lead Count Package Type = CQ Ceramic Quad Flat Pack = CG Ceramic Column Grid Aray = CC Ceramic Chip Carrier Land Grid Speed Grade Blank = Standard Speed 1 = Approximately 15% Faster than Standard Part Number RTSX32SU = 32,000 RadTolerant Typical Gates RTSX72SU = 72,000 RadTolerant Typical Gates RTSX-SU Device Status RTSX-SU Devices Status RTSX32SU Production RTSX72SU Production ii Revision 9 256 RTSX-SU Radiation-Tolerant FPGAs (UMC) Ceramic Device Resources User I/Os (including clock buffers) Device CQ84CQ208CQ256CC256CG624 RTSX32SU 62 173 227 202 – RTSX72SU – 170 212 – 360 Temperature Grade and Application Offering Package RTSX32SU RTSX72SU CQ84 B, E, EV, PROTO – CQ208 B, E, EV, PROTO B, E, EV, PROTO CQ256 B, E, EV, PROTO B, E, EV, PROTO CC256 M, B, E, EV, PROTO – CG624 – B, E, EV, PROTO Note: M = Military Temperature B = MIL-STD-883 Class B E = E-Flow EV = Microsemi SoC Products Group V Equivalent Flow (Class V Processing Consistent with MIL-PRF-38535) PROTO = RTSX-SU prototype units. Speed Grade and Temperature Grade Matrix RTSX32SU RTSX72SU STD ✓✓ –1 ✓✓ Notes: 1. Data applies to M, B, E, EV, and PROTO flow devices. 2. Contact your Microsemi SoC Products Group representative for availability. Revision 9 iii RTSX-SU Radiation-Tolerant FPGAs (UMC) QML Certification Microsemi has achieved full QML certification, demonstrating that quality management procedures, processes, and controls are in place and comply with MIL-PRF-38535 (the performance specification used by the U.S. Department of Defense for monolithic integrated circuits). MIL-STD-883 Class B Product Flow Table 2 • MIL-STD-883 Class B Product Flow* for RTSX32SU and RTSX72SU 883–Class B Step Screen 883 Method Requirement 1 Internal Visual 2010, Test Condition B 100% 2 Temperature Cycling 1010, Test Condition C 100% 3 Constant Acceleration 2001, Test Condition B or D, Y , Orientation Only 100% 1 4 Particle Impact Noise Detection 2020, Condition A 100% 5Seal 1014 a. Fine 100% b. Gross 100% 6 Visual Inspection 2009 100% 7 Pre-Burn-In In accordance with applicable Microsemi 100% Electrical Parameters device specification 8 Dynamic Burn-In 1015, Condition D, 100% 160 hours at 125°C or 80 hours at 150°C 9 Interim (Post-Burn-In) In accordance with applicable Microsemi 100% Electrical Parameters device specification 10 Percent Defective Allowable 5% All Lots 11 Final Electrical Test In accordance with applicable Microsemi a. Static Tests device specification, which includes a, b, and 100% (1) 25°C (Subgroup 1, Table I) 5005 (2) –55°C and +125°C (Subgroups 2, 3, Table I) 5005 b. Functional Tests 100% (1) 25°C (Subgroup 7, Table I) 5005 (2) –55°C and +125°C (Subgroups 8A and 8B, Table 5005 I) 100% c. Switching Tests at 25°C 5005 (Subgroup 9, Table I) 12. External Visual 2009 100% Note: *For CCGA devices, all Assembly, Screening, and TCI testing are performed at LGA level. Only QA electrical and mechanical visual are performed after solder column attachment. iv Revision 9 RTSX-SU Radiation-Tolerant FPGAs (UMC) Extended Flow 1,2 Table 3 • Extended Flow for RTSX32SU and RTSX72SU Step Screen Method Requirement 3 1 Destructive In-Line Bond Pull 2011, Condition D Sample 2 Internal Visual 2010, Condition A 100% 3 Serialization 100% 4 Temperature Cycling 1010, Condition C 100% 5 Constant Acceleration 2001, Condition B or D, Y1 Orientation Only 100% 6 Particle Impact Noise Detection 2020, Condition A 100% 6 7 Radiographic (X-Ray) 2012, One View (Y1 Orientation) Only 100% 8 Pre-Burn-In Test In accordance with applicable Microsemi device specification 100% 9 Dynamic Burn-In 1015, Condition D, 240 hours at 125°C or 120 hours at 150°C 100% minimum 10 Interim (Post-Burn-In) Electrical Parameters In accordance with applicable Microsemi device specification 100% 11 Static Burn-In 1015, Condition C, 72 hours at 150°C or 144 hours at 125°C 100% minimum 12 Interim (Post-Burn-In) Electrical Parameters In accordance with applicable Microsemi device specification 100% 13 Percent Defective Allowable (PDA) 5%, 3% Functional Parameters at 25°C All Lots Calculation 14 Final Electrical Test In accordance with Microsemi applicable device specification 100% which includes a, b, and c: a. Static Tests 100% (1) 25°C 5005 (Subgroup 1, Table1) (2) –55°C and +125°C 5005 (Subgroups 2, 3, Table 1) b. Functional Tests 100% (1) 25°C 5005 (Subgroup 7, Table 15) (2) –55°C and +125°C 5005 (Subgroups 8A and B, Table 1) c. Switching Tests at 25°C 100% (Subgroup 9, Table 1) 5005 15 Seal 1014 100% a. Fine b. Gross 16 External Visual 2009 100% Notes: 1. Microsemi offers Extended Flow for users requiring additional screening beyond MIL-STD-833, Class B requirement. Microsemi offers this Extended Flow incorporating the majority of the screening procedures as outlined in Method 5004 of MIL-STD-883, Class S. The exceptions to Method 5004 are shown in notes 2 and 4 below. 2. For CCGA devices, all Assembly/Screening/TCI testing are performed at LGA level. Only QA electrical and mechanical visual are performed after solder column attachment. 3. Method 5004 requires a 100 percent, nondestructive bond-pull to Method 2003. Microsemi substitutes a destructive bond-pull to Method 2011 Condition D on a sample basis only. 4. MIL-STD-883, Method 5004, requires a 100 percent radiation latch-up testing to Method 1020. Microsemi will NOT perform any radiation testing, and this requirement must be waived in its entirety. 5. Wafer lot acceptance complies to commercial standards only (requirement per Method 5007 is not performed). 6. X-Ray inspection is not performed on RTSX72SU in CQ208 and CQ256 packages because these packages contain a heat- spreader that prevents the X-Rays from penetrating through. Revision 9 v RTSX-SU Radiation-Tolerant FPGAs (UMC) EV Flow (Class V Flow Equivalent Processing) 1, 2, 3 Table 4 • EV Flow (Class V Equivalent Flow Processing) for RTSX32SU and RTSX72SU Step Screen Method Requirement 1 Destructive Bond Pull 2011, Condition D Extended Sample 2 Internal Visual 2010, Condition A 100% 3Serialization 100% 4 Temperature Cycling 1010, Condition C, 50 cycles minimum 100% 5 Constant Acceleration 2001, Y1 Orientation Only 100% 2001, Test Condition B or D, Y1, Orientation Only 6 Particle Impact Noise Detection 2020, Condition A 100% 4 7 Radiographic (X-Ray) 2012, One View (Y1 Orientation) Only 100% 8 Pre-Burn-In Electrical Parameters In accordance with applicable Microsemi device 100% specification 9 Dynamic Burn-In 1015, Condition D, 100% 240 hours at 125°C or 120 hours at 150°C minimum 10 Interim (Post-Dynamic-Burn-In) Electrical In accordance with applicable Microsemi device 100% Parameters specification 11 Static Burn-In 1015, Condition C, 72 hours at 150°C or 144 100% hours at 125°C minimum 12 Interim (Post-Static-Burn-In) Electrical In accordance with applicable Microsemi device 100% Parameters specification 13 Percent Defective Allowable (PDA) Calculation 5% Overall, 3% Functional Parameters at 25°C All Lots 14 Final Electrical Test In accordance with applicable Microsemi device 100% specification, which includes a, b, and c: a. Static Tests 5005, Table 1, Subgroup 1 (1) 25°C 5005, Table 1, Subgroup 2, 3 (2) –55°C and +125°C b. Functional Tests 5005, Table 1, Subgroup 7 (1) 25°C 5005, Table 1, Subgroup 8a, 8b (2) –55°C and +125°C 5005, Table 1, Subgroup 9 c. Switching Tests at 25°C 15 Seal (Fine & Gross Leak Test) 1014 100% 16 External Visual 2009 100% 17 Wafer Lot Specific Life Test (Group C) MIL-PRF-38535, Appendix B, sec. B.4.2.c All Wafer Lots Notes: 1. Microsemi offers EV flow for users requiring full compliance to MIL-PRF-38535 class V requirement. The EV process flow is expanded from the existing E-flow requirement (it still meets the full SMD requirement for current E-flow devices) with the intention to be in full compliance to MIL-PRF-38535 Table IA and Appendix B requirement, but without the official class V certification from DSCC. 2. For CCGA devices, all Assembly/Screening/TCI testing are performed at LGA level. Only QA electrical and mechanical visual are performed after solder column attachment. 3. As an enhancement to the Au ball bonding process control, two set-up units are required to go through 300°C bake for 60 minutes prior to the wire pull test. The minimum bond strength limit is 3.0 grams force, with no Au ball Lift mode (Au ball-to-Al pad separation) allowed regardless of bond strength. 4. X-Ray inspection is not performed on RTSX72SU in CQ208 and CQ256 packages because these packages contain a heat- spreader that prevents the X-Rays from penetrating through. vi Revision 9 RTSX-SU Radiation-Tolerant FPGAs (UMC) Table of Contents General Description Device Architecture . . . . . . . . . . . . . . . . . . . . . . . . . . . . . . . . . . . . . . . . . . . . . . . . . . . . . . . . . . . . . . . . . . . . . . . . . . 1-1 Programmable Interconnect Elements . . . . . . . . . . . . . . . . . . . . . . . . . . . . . . . . . . . . . . . . . . . . . . . . . . . . . . . . . . . 1-1 I/O Structure . . . . . . . . . . . . . . . . . . . . . . . . . . . . . . . . . . . . . . . . . . . . . . . . . . . . . . . . . . . . . . . . . . . . . . . . . . . . . . . 1-2 Logic Modules . . . . . . . . . . . . . . . . . . . . . . . . . . . . . . . . . . . . . . . . . . . . . . . . . . . . . . . . . . . . . . . . . . . . . . . . . . . . . . 1-3 Global Resources . . . . . . . . . . . . . . . . . . . . . . . . . . . . . . . . . . . . . . . . . . . . . . . . . . . . . . . . . . . . . . . . . . . . . . . . . . . 1-6 Design Environment . . . . . . . . . . . . . . . . . . . . . . . . . . . . . . . . . . . . . . . . . . . . . . . . . . . . . . . . . . . . . . . . . . . . . . . . . 1-6 Programming . . . . . . . . . . . . . . . . . . . . . . . . . . . . . . . . . . . . . . . . . . . . . . . . . . . . . . . . . . . . . . . . . . . . . . . . . . . . . . . 1-6 Low-Cost Prototyping Solution . . . . . . . . . . . . . . . . . . . . . . . . . . . . . . . . . . . . . . . . . . . . . . . . . . . . . . . . . . . . . . . . . 1-7 In-System Diagnostic and Debug Capabilities . . . . . . . . . . . . . . . . . . . . . . . . . . . . . . . . . . . . . . . . . . . . . . . . . . . . . . 1-7 Radiation Survivability . . . . . . . . . . . . . . . . . . . . . . . . . . . . . . . . . . . . . . . . . . . . . . . . . . . . . . . . . . . . . . . . . . . . . . . . 1-8 Summary . . . . . . . . . . . . . . . . . . . . . . . . . . . . . . . . . . . . . . . . . . . . . . . . . . . . . . . . . . . . . . . . . . . . . . . . . . . . . . . . . . 1-8 Related Documents . . . . . . . . . . . . . . . . . . . . . . . . . . . . . . . . . . . . . . . . . . . . . . . . . . . . . . . . . . . . . . . . . . . . . . . . . . 1-9 Detailed Specifications General Conditions . . . . . . . . . . . . . . . . . . . . . . . . . . . . . . . . . . . . . . . . . . . . . . . . . . . . . . . . . . . . . . . . . . . . . . . 2-1 Operating Conditions . . . . . . . . . . . . . . . . . . . . . . . . . . . . . . . . . . . . . . . . . . . . . . . . . . . . . . . . . . . . . . . . . . . . . . . . . 2-2 Thermal Characteristics . . . . . . . . . . . . . . . . . . . . . . . . . . . . . . . . . . . . . . . . . . . . . . . . . . . . . . . . . . . . . . . . . . . . . . . 2-7 Timing Model . . . . . . . . . . . . . . . . . . . . . . . . . . . . . . . . . . . . . . . . . . . . . . . . . . . . . . . . . . . . . . . . . . . . . . . . . . 2-10 I/O Specifications . . . . . . . . . . . . . . . . . . . . . . . . . . . . . . . . . . . . . . . . . . . . . . . . . . . . . . . . . . . . . . . . . . . . . . . . . . . 2-11 Module Specifications . . . . . . . . . . . . . . . . . . . . . . . . . . . . . . . . . . . . . . . . . . . . . . . . . . . . . . . . . . . . . . . . . . . . . . . 2-30 Routing Specifications . . . . . . . . . . . . . . . . . . . . . . . . . . . . . . . . . . . . . . . . . . . . . . . . . . . . . . . . . . . . . . . . . . . . . . . 2-35 Global Resources . . . . . . . . . . . . . . . . . . . . . . . . . . . . . . . . . . . . . . . . . . . . . . . . . . . . . . . . . . . . . . . . . . . . . . . . . . 2-37 Other Architectural Features . . . . . . . . . . . . . . . . . . . . . . . . . . . . . . . . . . . . . . . . . . . . . . . . . . . . . . . . . . . . . . . . . . 2-45 Package Pin Assignments CQ84 . . . . . . . . . . . . . . . . . . . . . . . . . . . . . . . . . . . . . . . . . . . . . . . . . . . . . . . . . . . . . . . . . . . . . . . . . . . . . . . . . . . . 3-1 CQ208 . . . . . . . . . . . . . . . . . . . . . . . . . . . . . . . . . . . . . . . . . . . . . . . . . . . . . . . . . . . . . . . . . . . . . . . . . . . . . . . . . . . . 3-3 CQ256 . . . . . . . . . . . . . . . . . . . . . . . . . . . . . . . . . . . . . . . . . . . . . . . . . . . . . . . . . . . . . . . . . . . . . . . . . . . . . . . . . . . . 3-7 CC256 . . . . . . . . . . . . . . . . . . . . . . . . . . . . . . . . . . . . . . . . . . . . . . . . . . . . . . . . . . . . . . . . . . . . . . . . . . . . . . . . . . . 3-12 CG624 . . . . . . . . . . . . . . . . . . . . . . . . . . . . . . . . . . . . . . . . . . . . . . . . . . . . . . . . . . . . . . . . . . . . . . . . . . . . . . . . . . . 3-21 Datasheet Information List of Changes . . . . . . . . . . . . . . . . . . . . . . . . . . . . . . . . . . . . . . . . . . . . . . . . . . . . . . . . . . . . . . . . . . . . . . . . . . . . . 4-1 Datasheet Categories . . . . . . . . . . . . . . . . . . . . . . . . . . . . . . . . . . . . . . . . . . . . . . . . . . . . . . . . . . . . . . . . . . . . . . . . 4-5 Export Administration Regulations (EAR) or International Traffic in Arms Regulations (ITAR) . . . . . . . . . . . . . . . . . 4-5 Revision 9 I 1 – General Description RTSX-SU radiation-tolerant FPGAs are enhanced versions of Microsemi’s SX-A family of devices, specifically designed for enhanced radiation performance. Featuring SEU-hardened D-type flip-flops that offer the benefits of Triple Module Redundancy (TMR) without the associated overhead, the RTSX-SU family is a unique product offering for space applications. Manufactured using 0.25 µm technology at the United Microelectronics Corporation (UMC) facility in Taiwan, RTSX-SU offers levels of radiation survivability far in excess of typical CMOS devices. Device Architecture RTSX-SU architecture, derived from the highly successful SX-A sea-of-modules architecture, has been designed to improve upset and total-dose performance in radiation environments. With three layers of metal interconnect in the RTSX32SU and four metal layers in RTSX72SU, the RTSX- SU family provides efficient use of silicon by locating the routing interconnect resources between the top two metal layers. This completely eliminates the channels of routing and interconnect resources between logic modules as found in traditional FPGAs. In a sea-of-modules architecture, the entire floor of the FPGA is covered with a grid of logic modules with virtually no chip area lost to interconnect elements or routing. The RTSX-SU architecture adds several enhancements over the SX-A architecture to improve its performance in radiation environments, such as SEU-hardened flip-flops, wider clock lines, and stronger clock drivers. Programmable Interconnect Elements Interconnection between logic modules is achieved using Microsemi’s patented metal-to-metal programmable antifuse interconnect elements. The antifuses are normally open circuit and form a permanent, low-impedance connection when programmed. The metal-to-metal antifuse is made up of a combination of amorphous silicon and dielectric material with barrier metals and has a programmed (“on” state) resistance of 25 Ω with capacitance of 1.0 fF for low signal impedance (Figure 1-1 on page 1-2). Revision 9 1-1 General Description Routing Tracks Amorphous Silicon/ Dielectric Antifuse Tungsten Plug Via Metal 4 Metal 3 Tungsten Plug Via Metal 2 Metal 1 Tungsten Plug Contact Silicon Substrate Figure 1-1 • RTSX-SU Family Interconnect Elements These antifuse interconnects reside between the top two layers of metal and thereby enable the sea-of- modules architecture in an FPGA. The extremely small size of these interconnect elements gives the RTSX-SU family abundant routing resources and provides excellent protection against design theft. Reverse engineering is virtually impossible because it is extremely difficult to distinguish between programmed and unprogrammed antifuses. Additionally, since RTSX-SU is a nonvolatile, single-chip solution, there is no configuration bitstream to intercept. The RTSX-SU interconnect (i.e., the antifuses and metal tracks) also has lower capacitance and resistance than that of any other device of similar capacity, leading to the fastest signal propagation in the industry for the radiation tolerance offered. I/O Structure The RTSX-SU family features a flexible I/O structure that supports 3.3 V LVTTL, 5 V TTL, 5 V CMOS, and 3.3 V and 5 V PCI. All I/O standards are hot-swap compliant, cold-sparing capable, and 5 V tolerant (except for 3.3 V PCI). In addition, each I/O on an RTSX-SU device can be configured as an input, an output, a tristate output, or a bidirectional pin. Mixed I/O standards are allowed and can be set on a pin-by-pin basis. High or low slew rate can be set on individual output buffers (except for PCI, which defaults to high slew), as well as the power-up configuration (either pull-up or pull-down). Even without the inclusion of dedicated I/O registers, these I/Os, in combination with array registers, can achieve clock-to-output-pad timing as fast as 9.5 ns. In most FPGAs, I/O cells that have embedded latches and flip-flops require instantiation in HDL code; this is a design complication not encountered in RTSX-SU FPGAs. Fast pin-to-pin timing ensures that the device will have little trouble interfacing with any other device in the system, which in turn, enables parallel design of system components and reduces overall design time. 1-2 Revision 9 RTSX-SU Radiation-Tolerant FPGAs (UMC) Logic Modules The RTSX-SU family provides two types of logic modules to the designer (Figure 1-2 on page 1-4): the register cell (R-cell) and the combinatorial cell (C-cell). The C-cell implements a range of combinatorial functions with up to five inputs. Inclusion of the DB input and its associated inverter function dramatically increases the number of combinatorial functions that can be implemented in a single module from 800 options (as in previous architectures) to more than 4,000 in the RTSX-SU architecture. An example of the improved flexibility enabled by the inversion capability is the ability to integrate a three-input exclusive-OR function into a single C-cell. This facilitates the construction of nine-bit parity-tree functions. At the same time, the C-cell structure is extremely synthesis-friendly, simplifying the overall design and reducing synthesis time. The R-cell contains a flip-flop featuring asynchronous clear, asynchronous preset, and clock enable (using the S0 and S1 lines) control signals. The R-cell registers feature programmable clock polarity, selectable on a register-by-register basis. This provides additional flexibility during mapping of synthesized functions into the RTSX-SU FPGA. The clock source for the R-cell can be chosen from the hardwired clock, the routed clocks, or the internal logic. While each SEU-hardened R-cell appears as a single D-type flip-flop to the user, each is implemented 2 employing triple redundancy to achieve a LET threshold of greater than 40 MeV-cm /mg. Each TMR R- cell consists of three master-slave latch pairs, each with asynchronous, self-correcting feedback paths. The output of each latch on the master or slave side is voted with the outputs of the other two latches on that side. If one of the three latches is struck by an ion and starts to change state, the voting with the other two latches prevents the change from feeding back and permanently latching. Care was taken in the layout to ensure that a single ion strike could not affect more than one latch (see the "R-Cell" section on page 2-31 for more details). Microsemi has arranged all C-cell and R-cell logic modules into horizontal banks called Clusters. There are two types of clusters: Type 1 contains two C-cells and one R-cell, while Type 2 contains one C-cell and two R-cells. To increase design efficiency and device performance, Microsemi has further organized these modules into SuperClusters. SuperCluster 1 is a two-wide grouping of Type 1 clusters. SuperCluster 2 is a two- wide group containing one Type 1 cluster and one Type 2 cluster. RTSX-SU devices feature more SuperCluster 1 modules than SuperCluster 2 modules because designers typically require significantly more combinatorial logic than flip-flops (Figure 1-2 on page 1-4). Revision 9 1-3 General Description Routing R-cells and C-cells within Clusters and SuperClusters can be connected through the use of two innovative local routing resources called FastConnect and DirectConnect, which enable extremely fast and predictable interconnection of modules within Clusters and SuperClusters. This routing architecture also dramatically reduces the number of antifuses required to complete a circuit, ensuring the highest possible performance (Figure 1-3 and Figure 1-4). DirectConnect is a horizontal routing resource that provides connections from a C-cell to its neighboring R-cell in a given SuperCluster. DirectConnect uses a hardwired signal path requiring no programmable interconnection to achieve its fast signal propagation time of less than 0.1 ns. FastConnect enables horizontal routing between any two logic modules within a given SuperCluster and vertical routing with the SuperCluster immediately below it. Only one programmable connection is used in a FastConnect path, delivering a maximum interconnect propagation delay of 0.4 ns. In addition to DirectConnect and FastConnect, the architecture makes use of two globally-oriented routing resources known as segmented routing and high-drive routing. Microsemi’s segmented routing structure provides a variety of track lengths for extremely fast routing between SuperClusters. The exact combination of track lengths and antifuses within each path is chosen by the 100-percent-automatic place-and-route software to minimize signal propagation delays. C-Cell R-Cell Routed D0 Data Input S1 D1 S0 PRE Y D2 Direct D3 DQ Y Connect Input Sa Sb HCLK CLKA, CLR DB CLKB, Internal Logic CKS CKP A0 B0 A1 B1 Cluster 1 Cluster 1 Cluster 2 Cluster 1 Type 1 SuperCluster Type 2 SuperCluster Figure 1-2 • R-Cell, C-Cell and Cluster Organization 1-4 Revision 9 RTSX-SU Radiation-Tolerant FPGAs (UMC) DirectConnect • No antifuses for smallest routing delay FastConnect • One antifuse Routing Segments • Typically 2 antifuses • Max. 5 antifuses Type 1 SuperClusters Figure 1-3 • DirectConnect and FastConnect for SuperCluster 1’s DirectConnect • No antifuses for smallest routing delay FastConnect • One antifuse Routing Segments • Typically 2 antifuses • Max. 5 antifuses Type 2 SuperClusters Figure 1-4 • DirectConnect and FastConnect for SuperCluster 2’s Revision 9 1-5 General Description Global Resources Microsemi’s high-drive routing structure provides three clock networks: hardwired clocks (HCLK), routed clocks (CLKA, CLKB), and quadrant clocks (QCLKA, QCLKB, QCLKC, QCLKD) (Table 1-1). Table 1-1 • RTSX-SU Global Resources RTSX32SU RTSX72SU Routed Clocks (CLKA, CLKB) 2 2 Hardwired Clocks (HCLK) 1 1 Quadrant Clocks (QCLKA, QCLKB, QCLKC, QCLKD) 0 4 The first clock, called HCLK, is hardwired from the HCLK buffer to the clock select MUX in each R-cell. HCLK cannot be connected to combinational logic. This provides a fast propagation path for the clock signal, enabling the 9.5 ns clock-to-out (pad-to-pad) performance of the RTSX-SU devices. The second type of clock, routed clocks (CLKA, CLKB), are global clocks that can be sourced from either external pins or internal logic signals within the device. CLKA and CLKB may be connected to sequential cells (R-cells) or to combinational logic (C-cells). The last type of clock, quadrant clocks, are only found in the RTSX72SU. Similar to the routed clocks, the four quadrant clocks (QCLKA, QCLKB, QCLKC, QCLKD) can be sourced from external pins or from internal logic signals within the device. Each of these clocks can individually drive up to a quarter of the chip, or they can be grouped together to drive multiple quadrants. Design Environment ® The RTSX-SU radiation-tolerant family of FPGAs is fully supported by both Libero Integrated Design Environment (IDE) software and Designer FPGA development software. Libero IDE is a design management environment, seamlessly integrating design tools while guiding the user through the design flow, managing all design and log files, and passing necessary design data among tools. Additionally, Libero IDE allows users to integrate both schematic and HDL synthesis into a single flow and verify the ® ® entire design in a single environment. Libero IDE includes Synplify from Synplicity , ViewDraw from ® Mentor Graphics, ModelSim™ HDL Simulator from Mentor Graphics , WaveFormer Lite™ from SynaptiCAD™, and Designer software from Microsemi. Refer to the Libero flow (located on the Microsemi SoC Products Group website) diagram for more information. Designer software is a place-and-route tool and provides a comprehensive suite of backend support tools for FPGA development. The Designer software includes timing-driven place-and-route, and a world-class integrated static timing analyzer and constraints editor. With the Designer software, a user can select and lock package pins while only minimally impacting the results of place-and-route. Additionally, the back-annotation flow is compatible with all the major simulators and the simulation results can be cross-probed with Silicon Explorer II, Microsemi’s integrated verification and logic analysis tool. Another tool included in the Designer software is the SmartGen core generator, which easily creates popular and commonly used logic functions for implementation into your schematic or HDL design. Designer software is compatible with the most popular FPGA design entry and verification tools from ® , and Cadence Design Systems. The companies such as Mentor Graphics, Synplicity, Synopsys Designer software is available for both the Windows and UNIX operating systems. Programming Programming support is provided through Microsemi's Silicon Sculptor series programmers, single-site programmers driven via a PC-based GUI. Factory programming is available as well. 1-6 Revision 9 RTSX-SU Radiation-Tolerant FPGAs (UMC) Low-Cost Prototyping Solution Since the enhanced radiation characteristics of radiation-tolerant devices are not required during the prototyping phase of the design, Microsemi has developed two prototyping solutions for RTSX-SU. For early design development and functional verification Microsemi offers the commercial SX-A devices, while for final flight design verification in hardware, Microsemi offers the RTSX-SU PROTO device that has the same fit, form and function as the flight silicon. Prototyping with SX-A Units The prototyping solution consists of two parts: • A well-documented design flow that allows the customer to target an RTSX-SU design to the equivalent commercial SX-A device • Either footprint-compatible packages or prototyping sockets to adapt commercial SX-A packages to the RTSX-SU package footprints This methodology provides the user with a cost-effective solution while maintaining the short time-to- market associated with Microsemi FPGAs. Please see the application note Prototyping for the RTSX-S Enhanced Aerospace FPGA for more details. Prototyping with RTSX-SU PROTO Units The RTSX-SU PROTO units offer a prototyping solution that can be used for final timing verification of the flight design. The RTSX-SU PROTO prototype units have the same timing attributes as the RTSX-SU flight units. Prototype units are offered in non-hermetic ceramic packages. The prototype units include "PROTO" in their part number, and "PROTO" is marked on devices to indicate that they are not intended for space flight. They also are not intended for applications, which require the quality of space-flight units, such as qualification of space-flight hardware. RT-PROTO units offer no guarantee of hermeticity, and no MIL-STD-883B processing. At a minimum, users should plan on using class B level devices for all qualification activities. The RT-PROTO units are electrically tested in a manner to guarantee their performance over the full military temperature range. The RT-PROTO units will also be offered in –1 or standard speed grades, so as to enable customers to validate the timing attributes of their space designs using actual flight silicon. In-System Diagnostic and Debug Capabilities The RTSX-SU family of FPGAs includes internal probe circuitry, allowing the designer to dynamically observe and analyze any signal inside the FPGA without disturbing normal device operation. Two individual signals can be brought out to two multipurpose pins (PRA and PRB) on the device. The probe circuitry is accessed and controlled via Silicon Explorer II, Microsemi's integrated verification and logic analysis tool, which attaches to the serial port of a PC and communicates with the FPGA via the JTAG port. Revision 9 1-7 General Description Figure 1-5 shows Silicon Explorer II and its connections. RTSX-SU FPGA TDI* TCK* Silicon Explorer II Serial Connection TMS* TDO* PRA* PRB* Note: *Refer to the "Pin Descriptions" section on page 2-11 for more information. Figure 1-5 • Probe Setup Radiation Survivability The RTSX-SU RadTolerant devices have varying total-dose radiation survivability. The ability of these devices to survive radiation effects is both device and lot dependent. Total-dose results are summarized in two ways. The first summary is indicated by the maximum total- dose level achieved before the device fails to meet an individual performance specification but remains functional. For Microsemi FPGAs, the parameter that first exceeds the specification is ICC (standby supply current). The second summary is indicated by the maximum total dose achieved prior to the functional failure of the device. Microsemi provides total-dose radiation test data on each lot. Reports are available on the Microsemi SoC Products Group website or from local sales representatives. Listings of available lots and devices can also be provided. For a radiation performance summary, see Radiation Data. This summary also shows single event upset (SEU) and single event latch-up (SEL) testing that has been performed on Microsemi FPGAs. All radiation performance information is provided for informational purposes only and is not guaranteed. Total dose effects are lot-dependent, and Microsemi does not guarantee that future devices will continue to exhibit similar radiation characteristics. In addition, actual performance can vary widely due to a variety of factors, including but not limited to, characteristics of the orbit, radiation environment, proximity to the satellite exterior, the amount of inherent shielding from other sources within the satellite, and actual bare die variations. For these reasons, it is the sole responsibility of the user to determine whether the device will meet the requirements of the specific design. Summary The RTSX-SU family of radiation-tolerant FPGAs extends Microsemi’s highly successful offering of FPGAs for radiation environments with the industry’s first FPGA designed specifically for enhanced radiation performance. 1-8 Revision 9 16 Additional Channels RTSX-SU Radiation-Tolerant FPGAs (UMC) Related Documents Application Notes Simultaneous Switching Noise and Signal Integrity http://www.microsemi.com/soc/documents/SSN_AN.pdf Implementation of Security in Actel Antifuse FPGAs http://www.microsemi.com/soc/documents/Antifuse_Security_AN.pdf Using A54SX72A and RT54SX72S Quadrant Clocks http://www.microsemi.com/soc/documents/QCLK_AN.pdf Actel eX, SX-A and RTSX-S I/Os http://www.microsemi.com/soc/documents/AntifuseIO_AN.pdf IEEE Standard 1149.1 (JTAG) in the SX/RTSX/SX-A/eX/RT54SX-S Families http://www.microsemi.com/soc/documents/SX_SXAJTAG_AN.pdf Prototyping for the RT54SX-S Enhanced Aerospace FPGA http://www.microsemi.com/soc/documents/RTSXS_Proto_AN.pdf Actel CQFP to FBFA Adapter Socket Instructions http://www.microsemi.com/soc/documents/CQ352-FPGA_Adapter_AN.pdf Actel SX-A and RT54SX-S Devices in Hot-Swap and Cold-Sparing Applications http://www.microsemi.com/soc/documents/HotSwapColdSparing_AN.pdf User’s Guides and Manuals Antifuse Macro Library Guide http://www.microsemi.com/soc/documents/libguide_ug.pdf SmartGen Core Reference Guide http://www.microsemi.com/soc/documents/gen_refguide_ug.pdf Libero SoC User's Guide http://www.microsemi.com/soc/documents/libero_ug.pdf Silicon Sculptor Software v5.0 for Silicon Sculptor II and Silicon Sculptor 3 Programmer User’s Guide http://www.microsemi.com/soc/documents/SiliSculptII_Sculpt3_ug.pdf Silicon Explorer User’s Guide http://www.microsemi.com/soc/documents/Silexpl_ug.pdf White Papers Design Security in Nonvolatile Flash and Antifuse FPGAs http://www.microsemi.com/soc/documents/DesignSecurity_WP.pdf Understanding Actel Antifuse Device Security http://www.microsemi.com/soc/documents/AntifuseSecurityWP.pdf Revision 9 1-9 2 – Detailed Specifications General Conditions Table 2-1 • Supply Voltages VCCA VCCI Maximum Input Tolerance Maximum Output Drive 2.5V 3.3V 5V* 3.3V 2.5V 5V 5V 5V Note: *3.3 V PCI is not 5 V tolerant Table 2-2 • Characteristics for All I/O Configurations I/O Standard Hot Swappable Slew Rate Control Power-Up Resistor* TTL, LVTTL Yes Yes. Affects falling edge outputs only Pull-up or Pull-down 3.3 V PCI No No. High slew rate only Pull-up or Pull-down 5 V PCI Yes No. High slew rate only Pull-up or Pull-down Note: *The pull-ups range from 25–35 Kohms and pull-downs range from 45–55 Kohm. The resistance values depend on VCCI, temperature, drive strength selection, and process. For board-level considerations and accurate modelling, use the corresponding IBIS models located on the Microsemi SoC Products Group website: http://www.microsemi.com/soc/download/ibis/milaero.aspx. Revision 9 2-1 Detailed Specifications Operating Conditions Absolute Maximum Conditions Stresses beyond those listed in Table 2-3 may cause permanent damage to the device. Exposure to absolute maximum rated conditions may affect device reliability. Devices should not be operated outside the recommendations in Table 2-4. 1 Table 2-3 • Absolute Maximum Conditions Symbol Parameter Limits Units T Junction Temperature –55 to 150 °C J 2 VCCA AC Core Supply Voltage –0.3 to 3.3 V VCCA DC Core Supply Voltage –0.3 to 3.0 V VCCI DC I/O Supply Voltage –0.3 to 6.0 V 3, 4 VI Input Voltage –0.5 to 6.0 V VI Input Voltage for PCI Inputs –0.5 to VCCI + 0.5 V VO Output Voltage –0.5 to 6.0 V T Storage Temperature –65 to 150 °C SG Notes: 1. Absolute maximum conditions are meant for short periods of transients only. They are not meant for operation of device for extended periods of time. 2. The AC transient VCCA limit is for radiation-induced transients less than 10 µs duration and not intended for repetitive use. Core voltage spikes from a single event transient will not negatively affect the reliability of the device if, for this non-repetitive event, the transient does not exceed 3.3 V at any time and the total time that the transient exceeds 2.75 V does not exceed 10 µs in duration. 3. For AC signals, the input signal may undershoot during transitions to –1.2 V for no longer than 11 ns. Current during the transition must not exceed 95 mA. 4. For AC signals, the 5 V non-PCI input signals may overshoot during transitions to VCCI + 1.2 V for no longer than 11 ns. The 3.3V non-PCI input signals may overshoot during transitions to 6.0 V for no longer than 11 ns. Current during the transition must not exceed 95 mA. Refer to PCI specifications for the PCI input overshoot limit. Table 2-4 • Recommended Operating Conditions Parameter Limits Units Temperature Range –55 to +125 °C 2.5 V Core Supply Voltage 2.25 to 2.75 V 3.3 V I/O Supply Voltage 3.0 to 3.6 V 5 V I/O Supply Voltage 4.5 to 5.5 V I (maximum) Standby Current (I + I)25 mA CC CCA CCI Power-Up and Power-Cycling The RTSX-SU family does not require any specific power-up or power-cycling sequence. 2-2 Revision 9 RTSX-SU Radiation-Tolerant FPGAs (UMC) Power Dissipation A critical element of system reliability is the ability of electronic devices to safely dissipate the heat generated during operation. The thermal characteristics of a circuit depend on the device and package used, the operating temperature, the operating current, and the system's ability to dissipate heat. A complete power evaluation should be performed early in the design process to help identify potential heat-related problems in the system and to prevent the system from exceeding the device’s maximum allowed junction temperature. The actual power dissipated by most applications is significantly lower than the power the package can dissipate. However, a thermal analysis should be performed for all projects. To perform a power evaluation, follow these steps: 1. Estimate the power consumption of the application. 2. Calculate the maximum power allowed for the device and package. 3. Compare the estimated power and maximum power values. Table 2-5 • RTSX-SU Standby Current Device Temperature ICCA (mA) ICCI (mA) RTSX72SU 25°C (typical) 3 7 125°C 10 15 RTSX32SU 25°C (typical) 1.5 7 125°C 5 15 Estimating Power Dissipation The total power dissipation for the RTSX-SU family is the sum of the DC power dissipation and the AC power dissipation: = PDC + PAC P Total EQ 1 DC Power Dissipation The power due to standby current is typically a small component of the overall power. The DC power dissipation is defined as: PDC = ICCA * VCCA + ICCI * VCCI EQ 2 Revision 9 2-3 Detailed Specifications AC Power Dissipation The power dissipation of the RTSX-SU family is usually dominated by the dynamic power dissipation. Dynamic power dissipation is a function of frequency, equivalent capacitance, and power supply voltage. The AC power dissipation is defined as follows: P = P + P + P + P + P + P + P AC(32SU) C-Cells R-Cells CLKA CLKB HCLK Output Buffer Input Buffer EQ 3 P = P + P + P + P + P + P + P AC(72SU) C-Cells R-Cells CLKA CLKB HCLK Output Buffer Input Buffer EQ 4 or: 2 P = VCCA * [(m * C * fm) + (m * C * fm) + (n * C * f ) + AC(32SU) EQCM C-Cells EQSM R-Cells EQI n Input Buffer (p * (C + C ) * f ) + (0.5 * (q * C * f ) + (r * f )) + EQO L p Output Buffer 1 EQCR q1 1 q1 CLKA (0.5 * (q * C * f ) + (r * f )) + (0.5 * (s * C * f ) + (C * f )) ] 2 EQCR q2 2 q2 CLKB 1 EQHV s1 EQHF s1 HCLK EQ 5 2 P = VCCA * [(m * C * fm ) + (m * C * fm ) + AC(72SU) c EQCM c C-Cells S EQSM S R-Cells (n * C * f ) + (0.5 * (q * C * f ) + (r * f )) + EQI n Input Buffer 1 EQCR q1 1 q1 RCLKA (0.5 * (q * C * f ) + (r * f )) + (0.5 * (s * C * f ) + (C * f ) 2 EQCR q2 2 q2 RCLKB 1 EQHV s1 EQHF s1 HCLK + (0.5 * (q * C * F ) + (r_cq * F )) + (0.5 * (q * C * F ) + cA EQCQ qcA qcA QCLKA cB EQCQ qcB (r_cq * F )) + (0.5* (q * C * F ) + (r_cq * F )) + qcB QCLKB cC EQCQ qcC qcC QCLKC (0.5 * (q * C * F ) + (r_cq * F ) ] + cD EQCQ qcD qcD QCLKD 2 VCCI * [(p * (C + CL) * f ) ] eqo p Output Buffer EQ 6 Where: = Equivalent capacitance of combinatorial modules (C-Cells) in pF C EQCM C = Equivalent capacitance of sequential modules (R-Cells) in pF EQSM C = Equivalent capacitance of input buffers in pF EQI C = Equivalent capacitance of output buffers in pF EQO C = Equivalent capacitance of CLKA/B in pF EQCR C = Variable capacitance of HCLK in pF EQHV C = Fixed capacitance of HCLK in pF EQHF C = Output load capacitance in pF L f = Average logic module switching rate in MHz m f = Average input buffer switching rate in MHz n f = Average output buffer switching rate in MHz p f = Average CLKA rate in MHz q1 f = Average CLKB rate in MHz q2 f = Average HCLK rate in MHz s1 F = Average QCLKA,QCLKB,QCLKC,QCLKD rate in MHz qcA,B,C,D m = Number of logic modules switching at fm n = Number of input buffers switching at fn 2-4 Revision 9 RTSX-SU Radiation-Tolerant FPGAs (UMC) p = Number of output buffers switching at fp q = Number of clock loads on CLKA 1 q = Number of clock loads on CLKB 2 qcA, qcB, qcC, qcD = Number of clock loads on QCLKA, QCLKB, QCLKC, QCLKD r = Fixed capacitance due to CLKA 1 r = Fixed capacitance due to CLKB 2 r_cq = Fixed capacitance due to QCLKA, QCLKB, QCLKC or QCLKD s Number of clock loads on HCLK 1 = x = Number of I/Os at logic low y = Number of I/Os at logic high Table 2-6 • Fixed Power Parameters Parameter RTSX32SU RTSX72SU Units C 3.00 3.00 pF EQCM C 3.00 3.00 pF EQSM C 1.40 1.30 pF EQI C 7.40 7.40 pF EQO C 3.50 3.50 pF EQCR C 4.30 4.30 pF EQHV C 300 690 pF EQHF C –3.5 pF EQCQ r 100 245 pF 1 r 100 245 pF 2 r_cq – 61.25 pF Revision 9 2-5 Detailed Specifications Guidelines for Estimating Power The following guidelines are meant to represent worst-case scenarios; they can be generally used to predict the upper limits of power dissipation: Logic Modules (m) = 20% of modules Inputs Switching (n) = # inputs/4 Outputs Switching (p) = # output/4 CLKA Loads (q1) = 20% of R-cells CLKB Loads (q2) = 20% of R-cells Load Capacitance (CL) = 35 pF Average Logic Module Switching Rate (fm) = f/10 Average Input Switching Rate (fn) =f/5 Average Output Switching Rate (fp) = f/10 Average CLKA Rate (fq1) = f/2 Average CLKB Rate (fq2) = f/2 Average HCLK Rate (fs1) = f HCLK loads (s1) = 20% of R-cells To assist customers in estimating the power dissipations of their designs, Microsemi has published the eX, SX-A and RT54SX-S Power Calculator worksheet. 2-6 Revision 9 RTSX-SU Radiation-Tolerant FPGAs (UMC) Thermal Characteristics Introduction The temperature variable in Microsemi’s Designer software refers to the junction temperature, not the ambient, case, or board temperatures. This is an important distinction because dynamic and static power consumption cause the chip junction to be higher than the ambient, case, or board temperatures. EQ 7, EQ 8, and EQ 9 give the relationship between thermal resistance, temperature gradient and power. T – T j a ----------------- θ = ja P EQ 7 T – T j c ----------------- θ = jc P EQ 8 T – T j b ----------------- θ = jb P EQ 9 Where: = Junction-to-air thermal resistance of the package. θ numbers are located in Table 2-7. θ ja ja θ = Junction-to-case thermal resistance of the package. θ numbers are located in jc jc Table 2-7. θ = Junction-to-board thermal resistance of the package. θ for a 624-pin CCGA is located jb jb in the notes for Table 2-7. T = Junction Temperature j T = Ambient Temperature a T = Board Temperature b T = Case Temperature c =Power P Revision 9 2-7 Detailed Specifications Package Thermal Characteristics The device thermal characteristics θ and θ are given in Table 2-7. The thermal characteristics for θ jc ja ja are shown with two different air flow rates. Note that the absolute maximum junction temperature is 150°C. Table 2-7 • Package Thermal Characteristics θ ja Pin Package Type Count θ Still Air θ 1.0m/s θ 2.5m/s Units jc ja ja 1 Ceramic Quad Flat Pack (CQFP) 84 2.0 40 33.0 30.0 °C/W 1 Ceramic Quad Flat Pack (CQFP) 208 2.0 22 19.8 18.0 °C/W 1 Ceramic Quad Flat Pack (CQFP) 256 2.0 20 16.5 15.0 °C/W 1 Ceramic Quad Flat Pack (CQFP) with 208 0.5 21.0 17.3 15.7 °C/W heatsink 1 Ceramic Quad Flat Pack (CQFP) with 256 0.5 19.0 15.7 14.2 °C/W heatsink 1 Ceramic Chip Carrier Land Grid (CCLG) 256 1.1 12.1 10.0 9.1 °C/W 2 Ceramic Column Grid Array (CCGA) 624 6.5 8.9 8.5 8.0 °C/W Notes: 1. θ for CQFP and CCLG packages refers to the thermal resistance between the junction and the bottom of jc the package. 2. θ for the CCGA 624 refers to the thermal resistance between the junction and the top surface of the jc package. Thermal resistance from junction to board (θ ) for CG624 package is 3.4 °C/W. jb Maximum Allowed Power Dissipation Shown below are example calculations to estimate the maximum allowed power dissipation for a given device based on two different thermal environments while maintaining the device junction temperature at or below worst-case military operating conditions (125°C). Example 1: This example assumes that there is still air in the environment. The heat flow is shown by the arrows in Figure 2-1 on page 2-8. The maximum ambient air temperature is assumed to be 50°C. The device package used is the 624-pin CCGA. Max. Junction Temp. – Max. Ambient Temp. 125°C – 50°C --------------------------------------------------------------------------------------------------------------------- ------------------------------------ Maximum Allowed Power == θ 8.9°C/W ja EQ 10 Air Solder Columns PCB Figure 2-1 • Hear Flow when Air is Present 2-8 Revision 9 RTSX-SU Radiation-Tolerant FPGAs (UMC) Example 2: This example assumes that the primary heat conduction path will be through the bottom of the package (neglecting the heat conducted through the package pins) to the board for a package mounted with thermal paste. The heat flow is shown by the arrows in Figure 2-2. The maximum board temperature is assumed to be 70°C. The device package used is the CQ352. The thermal resistance (θ ) of the thermal cb paste is assumed to be 0.58 °Χ/Ω. T – T T – T 125°C – 70°C j j j b Max. Allowed Power == --------------- ---------------------- = ------------------------------------------------------ = 21.32 W θ θ + θ 2.0°C/W + 0.58°C/W jb jc cb EQ 11 Thermal Adhesive PCB Figure 2-2 • Heat Flow in a Vacuum Timing Derating RTSX-SU devices are manufactured in a CMOS process; therefore, device performance is dependent on temperature, voltage, and process variations. Minimum timing parameters reflect maximum operating voltage, minimum operating temperature, and best-case processing. Maximum timing parameters reflect minimum operating voltage, maximum operating temperature, and worst-case processing. The derating factors shown in Table 2-8 should be applied to all timing data contained within this datasheet. Table 2-8 • Temperature and Voltage Derating Factors (Normalized to Worst-Case Military Conditions, T = 125°C, VCCA = 2.25 V) J Junction Temperature (T ) j VCCA –55°C –40°C 0°C 25°C 70°C 85°C 125°C 2.25 0.71 0.72 0.78 0.80 0.90 0.94 1.00 2.50 0.67 0.67 0.73 0.75 0.84 0.87 0.93 2.75 0.62 0.63 0.69 0.70 0.79 0.82 0.88 Note: The user can set the junction temperature in Microsemi’s Designer software to be any integer value in the range of –55°C to 175°C, and the core voltage to be any value between 2.25 V and 2.75 V. Revision 9 2-9 Detailed Specifications Timing Model Input Delays Internal Delays Predicted Output Delays Routing Delays Combinatorial t I/O Module = 0.8 ns I/O Module RD1 Cell t = 0.7 ns t = 1.0 ns INYH RD2 t = 3.8 ns t = 1.2 ns DHL PD t = 0.8 ns RD1 t = 1.5 ns RD4 t = 2.9 ns RD8 I/O Module t = 3.8 ns Register DHL Cell DQ t t = 0.8 ns = 0.8 ns SUD RD1 t = 0.0 ns HD t = 2.5 ns ENZL Routed t = 5.3 ns RCKH Clock t = 1.0 ns (100% Load) RCO I/O Module t = 3.8 ns Register DHL Cell I/O Module t = 0.7 ns INYH DQ t = 0.8 ns t = 0.8 ns SUD RD1 t = 0.0 ns t HD = 2.5 ns ENZL Hardwired Clock t t = 3.9 ns = 1.0 ns HCKH RCO Figure 2-3 • RTSX-SU Timing Model Values shown for RTSX32SU, –1, 0 krad (Si), 5 V TTL worst-case military conditions Table 2-9 • Hardwired Clock External Setup Clock-to-Out (pad-to-pad) = (t + t + t ) – t = t + t + t + t INYH RD2 SUD HCKH HCKH RCO RD1 DHL = 0.7 + 1.0 + 0.8 – 3.9 = –1.4 ns = 3.9 + 1.0 + 0.8 + 3.8 = 9.5 ns Table 2-10 • Routed Clock External Setup Clock-to-Out (pad-to-pad) = (t + t + t ) – t = t + t + t + t INYH RD2 SUD RCKH RCKH RCO RD1 DHL = 0.7 + 1.0 + 0.8 – 5.3= –2.8 ns = 5.3+ 1.0 + 0.8 + 3.8 = 10.9 ns 2-10 Revision 9 RTSX-SU Radiation-Tolerant FPGAs (UMC) I/O Specifications Pin Descriptions Supply Pins GND Ground Low supply voltage. VCCI Supply Voltage Supply voltage for I/Os. See Table 2-1 on page 2-1. VCCA Supply Voltage Supply voltage for Array. See Table 2-1 on page 2-1. Global Pins CLKA/B Routed Clock A and B These pins are clock inputs for clock distribution networks. Input levels are compatible with standard TTL, LVTTL, 3.3 V PCI, or 5 V PCI specifications. The clock input is buffered prior to clocking the R-cells. When not used, this pin must be set Low or High on the board. When used, this pin should be held Low or High during power-up to avoid unwanted static power. For RTSX72SU, these pins can be configured as user I/Os. When used, this pin offers a built-in programmable pull-up or pull-down resistor active during power-up only. QCLKA/B/C/D Quadrant Clock A, B, C, and D / I/O These four pins are the quadrant clock inputs and are only found on the RTSX72SU. They are clock inputs for clock distribution networks. Input levels are compatible with standard TTL, LVTTL, 3.3 V PCI or 5 V PCI specifications. Each of these clock inputs can drive up to a quarter of the chip, or they can be grouped together to drive multiple quadrants. The clock input is buffered prior to clocking the core cells. These pins can be configured as user I/Os. When not used, these pins must not be left floating. They must be set Low or High on the board. When used, these pins offer a built-in programmable pull-up or pull-down resistor, active during power-up only. HCLK Dedicated (Hardwired) Array Clock This pin is the clock input for sequential modules. Input levels are compatible with standard TTL, LVTTL, 3.3 V PCI or 5 V PCI specifications. This input is buffered prior to clocking the R-cells. It offers clock speeds independent of the number of R-cells being driven. When not used, this pin must not be left floating. It must be set to Low or High on the board. When used, this pin should be held Low or High during power-up to avoid unwanted static power. Revision 9 2-11 Detailed Specifications JTAG/Probe Pins 1 PRA/PRB , I/O Probe A/B The probe pin is used to output data from any user-defined design node within the device. This independent diagnostic pin can be used in conjunction with the other probe pin to allow real-time diagnostic output of any signal path within the device. The probe pin can be used as a user-defined I/O when verification has been completed. The pin’s probe capabilities can be permanently disabled to protect programmed design confidentiality. 1 TCK , I/O Test Clock Test clock input for diagnostic probe and device programming. In flexible mode, TCK becomes active when the TMS pin is set Low (Table 2-34 on page 2-45). This pin functions as an I/O when the boundary scan state machine reaches the “logic reset” state. 1 TDI , I/O Test Data Input Serial input for boundary scan testing and diagnostic probe. In flexible mode, TDI is active when the TMS pin is set Low (Table 2-34 on page 2-45). This pin functions as an I/O when the boundary scan state machine reaches the “logic reset” state. 2 TDO , I/O Test Data Output Serial output for boundary scan testing. In flexible mode, TDO is active when the TMS pin is set Low (Table 2-34 on page 2-45). This pin functions as an I/O when the boundary scan state machine reaches the logic reset state. When Silicon Explorer II is being used, TDO will act as an output when the checksum command is run. It will return to user I/O when checksum is complete. 2 TMS Test Mode Select The TMS pin controls the use of the IEEE 1149.1 boundary scan pins (TCK, TDI, TDO, TRST). In flexible mode when the TMS pin is set Low, the TCK, TDI, and TDO pins are boundary scan pins (Table 2-34 on page 2-45). Once the boundary scan pins are in test mode, they will remain in that mode until the internal boundary scan state machine reaches the “logic reset” state. At this point, the boundary scan pins will be released and will function as regular I/O pins. The “logic reset” state is reached five TCK cycles after the TMS pin is set High. In dedicated test mode, TMS functions as specified in the IEEE 1149.1 specifications. 1. Microsemi SoC Products Group recommends that you use a series termination resistor on every probe connector (TDI, TCK, TDO, PRA, and PRB). The series termination is used to prevent data transmission corruption (i.e., due to reflection from the FPGA to the probe connector) during probing and reading back the checksum. With an internal set-up we have seen 70-ohm termination resistor improved the signal transmission. Since the series termination depends on the setup, Microsemi SoC Products Group recommends users calculate the termination resistor for their own setup. Below is a guideline on how to calculate the resistor value. The resistor value should be chosen so that the sum of it and the probe signal’s driver impedance equals the effective trace impedance. Z0 = Rs + Zd Z0 = trace impedance (Silicon Explorer’s breakout cable’s resistance + PCB trace impedance), Rs= series termination, Zd= probe signal’s driver impedance. The termination resistor should be placed as close as possible to the driver. Among the probe signals, TDI, TCK, and TMS are driven by Silicon Explorer. A54SX16 is used in Silicon Explorer and hence the driver impedances needs to be calculated from the SX08/SX16/SX32 IBIS Model IBIS Model (Mixed Voltage Operation). PRA, PRB, and TDO are driven by the FPGA and driver impedance can also be calculated from the IBIS Model. Silicon Explorer’s breakout cable’s resistance is usually close to 1 ohm. 2. Microsemi SoC Products Group recommends that you use a series termination resistor on every probe connector (TDI, TCK, TDO, PRA, and PRB). The series termination is used to prevent data transmission corruption (i.e., due to reflection from the FPGA to the probe connector) during probing and reading back the checksum. With an internal set-up we have seen 70-ohm termination resistor improved the signal transmission. Since the series termination depends on the setup, Microsemi SoC Products Group recommends users to calculate the termination resistor for their own setup. Below is a guideline on how to calculate the resistor value. The resistor value should be chosen so that the sum of it and the probe signal’s driver impedance equals the effective trace impedance. Z0 = Rs + Zd Z0 = trace impedance (Silicon Explorer’s breakout cable’s resistance + PCB trace impedance), Rs= series termination, Zd= probe signal’s driver impedance. The termination resistor should be placed as close as possible to the driver. Among the probe signals, TDI, TCK, and TMS are driven by Silicon Explorer. A54SX16 is used in Silicon Explorer and hence the driver impedances needs to be calculated from the SX08/SX16/SX32 IBIS Model (Mixed Voltage Operation). PRA, PRB, and TDO are driven by the FPGA and driver impedance can also be calculated from the IBIS Model. Silicon Explorer’s breakout cable’s resistance is usually close to 1 ohm. 2-12 Revision 9 RTSX-SU Radiation-Tolerant FPGAs (UMC) TRST Boundary Scan Reset Pin The TRST pin functions as an active-low input to asynchronously initialize or rest the boundary scan circuit. The TRST pin is equipped with an internal pull-up resistor. For flight applications, the TRST pin should be hardwired to GND. User I/O I/O Input/Output The I/O pin functions as an input, output, tristate, or bidirectional buffer. Input and output levels are compatible with standard TTL, LVTTL, 3.3 V/5 V PCI, or 5 V CMOS specifications. Unused I/O pins are automatically tristated by the Designer software. Care must be exercised when using JTAG (HIGHZ command) to tristate all I/Os because the pull-ups and pull-downs will be enabled if programmed. See the "User I/O" section on page 2-13 for more details. Special Functions NC No Connection This pin is not connected to circuitry within the device. These pins can be driven to any voltage or can be left floating with no effect on the operation of the device. User I/O The RTSX-SU family features a flexible I/O structure that supports 3.3 V LVTTL, 5 V TTL, 5 V CMOS, and 3.3 V and 5 V PCI. All I/O standards are hot-swap compliant, cold-sparing capable, and 5V tolerant (except for 3.3 V PCI). Each I/O module has an available power-up resistor of approximately 50 kΩ that can configure the I/O to a known state during power-up. Just slightly before VCCA reaches 2.5 V, the resistors are disabled so the I/Os will behave normally. Note: Care must be exercised when using JTAG (HIGHZ command) to tristate all I/Os because the pull- ups and pull-downs will be enabled if programmed. For more information about the power-up resistors, refer to Microsemi’s application note Microsemi SX-A and RTSX-SU Devices in Hot-Swap and Cold-Sparing Applications. RTSX-SU inputs should be driven by high-speed push-pull devices with a low-resistance pull-up device. If the input voltage is greater than VCCI and a fast push-pull device is NOT used, the high-resistance pull-up of the driver and the internal circuitry of the RTSX-SU I/O may create a voltage divider (when a user I/O is configured as an input, the associated output buffer is tristated). This voltage divider could pull the input voltage below specification for some devices connected to the driver. A logic ‘1’ may not be correctly presented in this case. For example, if an open drain driver is used with a pull-up resistor to 5 V to provide the logic ‘1’ input, and VCCI is set to 3.3 V on the RTSX-SU device, the input signal may be pulled down by the RTSX-SU input. Hot Swapping RTSX-SU I/Os can be configured to be hot swappable in compliance with the Compact PCI Specification. However, 3.3 V PCI I/Os are not hot swappable. When the RTSX-SU FPGA is plugged into an electrically-active system, designers should wait for the device to complete its power-up initialization before expecting valid levels on the outputs. Refer to Microsemi’s application note, SX-A and RTSX-S Devices in Hot-Swap and Cold-Sparing Applications for more information on hot swapping. Customizing the I/O Each user I/O on an RTSX-SU device can be configured as an input, an output, a tristate output, or a bidirectional pin. Mixed I/O standards are allowed and can be set on a pin-by-pin basis. High or low slew rates can be set on individual output buffers (except for PCI which defaults to high slew), as well as the power-up configuration (either pull-up or pull-down). The user selects the desired I/O by setting the I/O properties in PinEditor, Microsemi’s graphical pin- placement and I/O properties editor. See the PinEditor online help for more information. Revision 9 2-13 Detailed Specifications Unused I/Os All unused user I/Os are automatically tristated by Microsemi’s Designer software. Although termination is not required, it is recommended that the user tie off all unused I/Os to GND externally. If the I/O clamp diode is disabled, then unused I/Os are 5 V tolerant, otherwise unused I/Os are tolerant to VCCI. Using the Weak Pull-Up and Pull-Down Circuits Each RTSX-SU I/O comes with a weak pull-up (25-35 Kohm range) and pull-down circuit (45-55 Kohm range). I/O macros are provided for various standards (TTL, CMOS) and can be instantiated if a pull-up/pull-down is required. The resistance values depend on VCCI, temperature, drive strength selection, and process. For board-level considerations and accurate modelling, use the corresponding IBIS models, located on the Microsemi SoC Products Group website at http://www.microsemi.com/soc/download/ibis/milaero.aspx. I/O Macros There are nine I/O macros available to the user for RTSX-SU: • CLKBUF/CLKBUFI: Clock Buffer, noninverting and inverting • CLKBIBUF/CLKBIBUFI: Bidirectional Clock Buffer, noninverting and inverting • QCLKBUF/QCLKBUFI: Quad Clock Buffer, noninverting and inverting • QCLKBIBUF/QCLKBIBUFI: Quad Bidirectional Clock Buffer, noninverting and inverting • HCLKBUF: Hardwired Clock Buffer • INBUF: Input Buffer • OUTBUF: Output Buffer • TRIBUF: Tristate Buffer • BIBUF: Bidirectional Buffer Table 2-11 • User I/O Features Function Description Input Buffer Threshold • 5 V: CMOS, PCI, TTL Selections • 3.3 V: PCI, LVTTL Flexible Output Driver • 5 V: CMOS, PCI, TTL • 3.3 V: PCI, LVTTL • Selectable on an individual I/O basis Output Buffer Hot-Swap Capability • I/Os on an unpowered device does not sink the current (Power supplies are at 0 V) • Can be used for cold sparing Individually selectable slew rate, high or low slew (The default is high slew rate). The slew rate selection only affects the falling edge of an output. There is no change on the rising edge of the output or any inputs Power-Up Individually selectable pull-ups and pull-downs during power-up (default is to power-up in tristate mode) Enables deterministic power-up of a device VCCA and VCCI can be powered in any order 2-14 Revision 9 RTSX-SU Radiation-Tolerant FPGAs (UMC) I/O Module Timing Characteristics E D TRIBUFF PAD To AC test loads (shown below) VCC VCC VCC GND D 50% 50% E 50% GND E 50% 50% 50% GND VOH VCC VOH V V MEAS 90% Pad MEAS Pad V 10% VPad MEAS V MEAS VOL VOL GND t t t t t t DLH DHL ENZL ENLZ ENZH ENHZ Figure 2-4 • Output Timing Model and Waveforms VCCI Pad V 0 V V MEAS MEAS VCC PAD Y INBUF 50% Y 50% GND t t INYH INYL Figure 2-5 • Input Timing Model and Waveforms Load 2 Load 3 Load 1 (Used to measure enable delays) (Used to measure disable delays) (Used to measure propagation delay) GND VCC GND VCC To the output under test R to VCC for t R to VCC for t 35 pF PZL PLZ R to GND for t R to GND for t PZH PHZ To the output To the output R = 1 kΩ R = 1 kΩ under test under test 35 pF 5 pF Figure 2-6 • AC Test Loads Revision 9 2-15 Detailed Specifications 5 V TTL and 3.3 V LVTTL Table 2-12 • 5 V TTL and 3.3 V LVTTL Electrical Specifications Military Symbol Parameter Min. Max. Units VOH VCCI = Min. (I = –1mA) 0.9 VCCI V OH VI = VIH or VIL VCCI = Min. (I = –8mA) 2.4 V OH VI = VIH or VIL VOL VCCI = Min. (I = 1mA) 0.1 VCCI V OL VI = VIH or VIL VCCI = Min. (I = 12mA) 0.4 V OL VI = VIH or VIL 1 VIL Input Low Voltage –0.5 0.8 V VIH Input High Voltage 2.0 V I / I Input Leakage Current, VIN = VCCI or GND (VCCI ≤ 5.25 V) –20 20 µA IL IH (VCCI ≤ 5.5 V) –70 70 µA I Tristate Output Leakage Current, VOUT = VCCI or (VCCI ≤ 5.25 V) –20 20 µA OZ GND (VCCI ≤ 5.5 V) –70 70 µA 2 t , t Input Transition Time 10 ns R F 3 C Input Pin Capacitance 20 pF IN 3 C CLK Pin Capacitance 20 pF CLK VMEAS Trip point for Input buffers and Measuring point for 1.5 V Output buffers 4 IV Curve Can be derived from the IBIS model on the web. Notes: 1. For AC signals, the input signal may undershoot during transitions to –1.2 V for no longer than 11 ns. Current during the transition must not exceed 95 mA. 2. If t or t exceeds the limit of 10 ns, Microsemi can guarantee reliability but not functionality. R F 3. Absolute maximum pin capacitance, which includes package and I/O input capacitance. 4. The IBIS model can be found at www.microsemi.com/soc/techdocs/models/ibis.html. 2-16 Revision 9 RTSX-SU Radiation-Tolerant FPGAs (UMC) Timing Characteristics Table 2-13 • RTSX32SU 5 V TTL and 3.3 V LVTTL I/O Module = 125°C Worst-Case Military Conditions VCCA = 2.25 V, T J Radiation Level = 0 krad (Si) –1 Speed Std. Speed Parameter Description Min. Max. Min. Max. Units 5 V TTL Output Module Timing (VCCI = 4.5 V) t Input Data Pad-to-Y High 0.7 0.9 ns INYH t Input Data Pad-to-Y Low 1.1 1.3 ns INYL t Data-to-Pad Low to High 3.1 3.6 ns DLH t Data-to-Pad High to Low 3.8 4.4 ns DHL t Data-to-Pad High to Low – low slew 9.8 11.5 ns DHLS t Enable-to-Pad, Z to Low 3.8 4.4 ns ENZL t Enable-to-Pad, Z to Low – low slew 9.8 11.5 ns DENZLS t Enable-to-Pad, Z to High 3.1 3.6 ns ENZH t Enable-to-Pad, Low to Z 3.1 3.6 ns ENLZ t Enable-to-Pad, High to Z 3.8 4.4 ns ENHZ t Enable-to-Pad, H to Z – low slew 9.8 11.5 ns ENZHS 2 d Delta Delay vs. Load Low to High 0.036 0.046 ns/pF TLH 2 d Delta Delay vs. Load High to Low 0.029 0.038 ns/pF THL 2 d Delta Delay vs. Load High to Low – low slew 0.049 0.064 ns/pF THLS 3.3 V LVTTL Output Module Timing (VCCI = 3.0 V) t Input Data Pad-to-Y High 0.8 0.9 ns INYH t Input Data Pad-to-Y Low 1.1 1.3 ns INYL t Data-to-Pad Low to High 4.1 4.8 ns DLH t Data-to-Pad High to Low 3.7 4.4 ns DHL t Data-to-Pad High to Low – low slew 13.2 15.6 ns DHLS t Enable-to-Pad, Z to L 3.7 4.4 ns ENZL t Enable-to-Pad, Z to Low – low slew 13.2 15.6 ns DENZLS t Enable-to-Pad, Z to H 4.1 4.8 ns ENZH t Enable-to-Pad, L to Z 4.1 4.8 ns ENLZ t Enable-to-Pad, H to Z 3.7 4.4 ns ENHZ t Enable-to-Pad, H to Z – low slew 13.2 15.6 ns ENZHS 2 d Delta Delay vs. Load Low to High 0.064 0.081 ns/pF TLH 2 d Delta Delay vs. Load High to Low 0.031 0.040 ns/pF THL 2 d Delta Delay vs. Load High to Low – low slew 0.069 0.088 ns/pF THLS Notes: 1. Output delays based on 35 pF loading. 2. To obtain the slew rate, substitute the appropriate Delta value, load capacitance, and the VCCI value into the following equation: Slew Rate [V/ns] = ±(0.1 * VCCI - 0.9 * VCCI)/ (C * d |d |d ) load TLH THL THLS where C is the load capacitance driven by the I/O in pF; load d |d |d is the worst case delta value from the datasheet in ns/pF. TLH THL THLS Revision 9 2-17 Detailed Specifications Table 2-14 • RTSX72SU 5 V TTL and 3.3 V LVTTL I/O Module Worst-Case Military Conditions VCCA = 2.25 V, T = 125°C J Radiation Level = 0 krad (Si) –1 Speed Std. Speed Parameter Description Min. Max. Min. Max. Units 5 V TTL Output Module Timing (VCCI = 4.5 V) t Input Data Pad-to-Y High 0.7 0.9 ns INYH t Input Data Pad-to-Y Low 1.1 1.3 ns INYL t Data-to-Pad Low to High 3.2 3.7 ns DLH t Data-to-Pad High to Low 4.0 4.7 ns DHL t Data-to-Pad High to Low – low slew 10.3 12.1 ns DHLS t Enable-to-Pad, Z to Low 4.0 4.7 ns ENZL t Enable-to-Pad, Z to Low – low slew 10.3 12.1 ns DENZLS t Enable-to-Pad, Z to High 3.2 3.7 ns ENZH t Enable-to-Pad, Low to Z 3.2 3.7 ns ENLZ t Enable-to-Pad, High to Z 4.0 4.7 ns ENHZ t Enable-to-Pad, High to Z – low slew 10.3 12.1 ns ENHZS 2 d Delta Delay vs. Load Low to High 0.036 0.046 ns/pF TLH 2 d Delta Delay vs. Load High to Low 0.029 0.038 ns/pF THL 2 d Delta Delay vs. Load High to Low – low slew 0.049 0.064 ns/pF THLS 3.3 V LVTTL Output Module Timing (VCCI = 3.0 V) t Input Data Pad-to-Y High 1.0 1.2 ns INYH t Input Data Pad-to-Y Low 2.2 2.5 ns INYL t Data-to-Pad Low to High 4.0 4.6 ns DLH t Data-to-Pad High to Low 3.6 4.2 ns DHL t Data-to-Pad High to Low – low slew 12.7 14.9 ns DHLS t Enable-to-Pad, Z to L 3.6 4.2 ns ENZL t Enable-to-Pad, Z to Low – low slew 12.7 14.9 ns DENZLS t Enable-to-Pad, Z to H 4.0 4.6 ns ENZH t Enable-to-Pad, L to Z 4.0 4.6 ns ENLZ t Enable-to-Pad, H to Z 3.6 4.2 ns ENHZ t Enable-to-Pad, High to Z – low slew 12.7 14.9 ENHZS 2 d Delta Delay vs. Load Low to High 0.064 0.081 ns/pF TLH 2 d Delta Delay vs. Load High to Low 0.031 0.04 ns/pF THL 2 d Delta Delay vs. Load High to Low – low slew 0.069 0.088 ns/pF THLS Notes: 1. Output delays based on 35 pF loading. 2. To obtain the slew rate, substitute the appropriate Delta value, load capacitance, and the VCCI value into the following equation: Slew Rate [V/ns] = ±(0.1 * VCCI - 0.9 * VCCI)/ (C * d |d |d ) load TLH THL THLS where C is the load capacitance driven by the I/O in pF; load d |d |d is the worst case delta value from the datasheet in ns/pF. TLH THL THLS 2-18 Revision 9 RTSX-SU Radiation-Tolerant FPGAs (UMC) 5V CMOS Table 2-15 • 5 V CMOS Electrical Specifications Military Symbol Parameter Min. Max. Units VOH VCCI = MIN, (I = –20 µA) VCCI – 0.1 V OH VI = VCCI or GND VOL VCCI = MIN, (I = ±20 µA) 0.1 V OL VI = VCCI or GND 1 VIL Input Low Voltage, VOUT = VOL (maximum) – 0.5 0.3 V V CC V Input High Voltage, VOUT = VOH (minimum) 0.7 VCC V IH I /I Input Leakage Current, VIN = VCCI or GND (VCCI ≤ 5.25 V) –20 20 µA IL IH (VCCI ≤ 5.5 V) –70 70 µA I Tristate Output Leakage Current, VOUT = VCCI or GND (VCCI ≤ 5.25 V) –20 20 µA OZ (VCCI ≤ 5.5 V) –70 70 µA t , t Input Transition Time 10 ns R F 2 C Input Pin Capacitance 20 pF IN 2 C CLK Pin Capacitance 20 pF CLK VMEAS Trip point for Input buffers and Measuring point for 2.5 V Output buffers IV Can be derived from the IBIS model on the web. 2 Curve Notes: 1. For AC signals, the input signal may undershoot during transitions –1.2 V for no longer than 11 ns. Current during the transition must not exceed 95 mA. 2. The IBIS model can be found at www.microsemi.com/soc/techdocs/models/ibis.html. Revision 9 2-19 Detailed Specifications Timing Characteristics Table 2-16 • RTSX32SU 5 V CMOS I/O Module = 125°C Worst-Case Military Conditions VCCA = 2.25 V, VCCI = 4.5 V, T J Radiation Level = 0 krad (Si) –1 Speed Std. Speed Parameter Description Min. Max. Min. Max. Units 5 V CMOS Output Module Timing t Input Data Pad-to-Y High 0.7 0.9 ns INYH t Input Data Pad-to-Y Low 1.1 1.3 ns INYL t Data-to-Pad Low to High 3.4 4.0 ns DLH t Data-to-Pad High to Low 3.6 4.2 ns DHL t Data-to-Pad High to Low – low slew 8.7 10.3 ns DHLS t Enable-to-Pad, Z to Low 3.4 4.0 ns ENZL t Enable-to-Pad, Z to Low – low slew 8.7 10.3 ns DENZLS t Enable-to-Pad, Z to High 3.6 4.2 ns ENZH t Enable-to-Pad, Low to Z 3.6 4.2 ns ENLZ t Enable-to-Pad, High to Z 3.4 4.0 ns ENHZ t Enable-to-Pad, High to Z — low slew 8.7 10.3 ns ENHZS 2 d Delta Delay vs. Load Low to High 8.68 10.21 ns/pF TLH 2 d Delta Delay vs. Load High to Low 0.029 0.038 ns/pF THL 2 d Delta Delay vs. Load High to Low – low slew 0.049 0.064 ns/pF THLS Notes: 1. Output delays based on 35 pF loading. 2. To obtain the slew rate, substitute the appropriate Delta value, load capacitance, and the VCCI value into the following equation: Slew Rate [V/ns] = ±(0.1 * VCCI - 0.9 * VCCI)/ (C * d |d |d ) load TLH THL THLS where C is the load capacitance driven by the I/O in pF; load d |d |d is the worst case delta value from the datasheet in ns/pF. TLH THL THLS 2-20 Revision 9 RTSX-SU Radiation-Tolerant FPGAs (UMC) Table 2-17 • RTSX72SU 5 V CMOS I/O Module Worst-Case Military Conditions VCCA = 2.25 V, VCCI = 4.5 V, T = 125°C J Radiation Level = 0 krad (Si) –1 Speed Std. Speed Parameter Description Min. Max. Min. Max. Units 5 V CMOS Output Module Timing t Input Data Pad-to-Y High 0.7 0.9 ns INYH t Input Data Pad-to-Y Low 1.1 1.3 ns INYL t Data-to-Pad Low to High 3.6 4.2 ns DLH t Data-to-Pad High to Low 3.8 4.5 ns DHL t Data-to-Pad High to Low – low slew 9.2 10.8 ns DHLS t Enable-to-Pad, Z to Low 3.6 4.2 ns ENZL t Enable-to-Pad, Z to Low – low slew 9.2 10.8 ns DENZLS t Enable-to-Pad, Z to High 3.8 4.5 ns ENZH t Enable-to-Pad, Low to Z 3.8 4.5 ns ENLZ t Enable-to-Pad, High to Z 3.6 4.2 ns ENHZ t Enable-to-Pad, High to Z – low slew 9.2 10.8 ns ENZHS 2 d Delta Delay vs. Load Low to High 0.036 0.046 ns/pF TLH 2 d Delta Delay vs. Load High to Low 0.029 0.038 ns/pF THL 2 d Delta Delay vs. Load High to Low – low 0.049 0.064 ns/pF THLS slew Notes: 1. Output delays based on 35 pF loading. 2. To obtain the slew rate, substitute the appropriate Delta value, load capacitance, and the VCCI value into the following equation: Slew Rate [V/ns] = ±(0.1* VCCI - 0.9 * VCCI)/ (C * d |d |d ) load TLH THL THLS where C is the load capacitance driven by the I/O in pF; load d |d |d is the worst case delta value from the datasheet in ns/pF. TLH THL THLS Revision 9 2-21 Detailed Specifications 5V PCI The RTSX-SU family supports 5 V PCI and is compliant with the PCI Local Bus Specification Rev. 2.1. Table 2-18 • 5 V PCI DC Specifications Symbol Parameter Condition Min. Max. Units VCCA Supply Voltage for Array 2.25 2.75 V VCCI Supply Voltage for I/Os 4.5 5.5 V 1 VIH Input High Voltage 2.0 VCCI + 0.5 V 1 VIL Input Low Voltage –0.5 0.8 V I Input High Leakage Current VIN = 2.75 70 µA IH I Input Low Leakage Current VIN = 0.5 –70 µA IL VOH Output High Voltage I = –2 mA 2.4 V OUT 2 VOL Output Low Voltage I = 3 mA, 6 mA 0.55 V OUT 3 C Input Pin Capacitance 10 pF IN C CLK Pin Capacitance 5 12 pF CLK VMEAS Trip Point for Input Buffers and Measuring Point for 1.5 V Output Buffers Notes: 1. Input leakage currents include hi-Z output leakage for all bidirectional buffers with tristate outputs. 2. Signals without pull-up resistors must have 3 mA low output current. Signals requiring pull-up must have 6 mA; the latter include, FRAME#, IRDY#, TRDY#, DEVSEL#, STOP#, SERR#, PERR#, LOCK#, and, when used AD[63:32], C/BE[7:4]#, PAR64, REQ64#, and ACK64#. 3. Absolute maximum pin capacitance for a PCI input is 10 pF (except for CLK) with an exception granted to motherboard- only devices, which could be up to 16 pF in order to accommodate PGA packaging. This mean that components for expansion boards need to use alternatives to ceramic PGA packaging (i.e., PBGA,PQFP, SGA, etc.). 4. For AC signals, the input signal may undershoot during transitions to –1.2 V for no longer than 11 ns. Current during the transition must not exceed 95 mA. 200.0 I Max. Specification OL I OL 150.0 100.0 I Min. Specification OL 50.0 0.0 0 0.5 1 1.5 2 2.5 3 3.5 4 4.5 5 5.5 6 –50.0 I Min. Specification OH I Max. Specification OH –100.0 –150.0 I OH –200.0 Voltage Out (V) Figure 2-7 • 5 V PCI V/I Curve for RTSX-SU 2-22 Revision 9 Current (mA) RTSX-SU Radiation-Tolerant FPGAs (UMC) Equation A I = 11.9 * (VOUT – 5.25) * (VOUT + 2.45) OH for VCCI > VOUT > 3.1 V Equation B I = 78.5 * VOUT * (4.4 – VOUT) OL for 0 V < VOUT < 0.71 V Table 2-19 • 5 V PCI AC Specifications Symbol Parameter Condition Min. Max. Units 1 0 < VOUT < 1.4 –44 mA I OH(AC) 1, 2 Switching Current High 1.4 < VOUT < 2.4 (–44 + (VOUT – 1.4)/0.024) mA 1, 3 3.1 < VOUT < VCCI "Equation A" 3 (Test Point) VOUT = 3.1 –142 mA 1 I VOUT = 2.2 95 mA OL(AC) 1 Switching Current Low 2.2 > VOUT > 0.55 (VOUT/0.023) mA 1, 3 0.71 > VOUT > 0 "Equation B" (Test Point) VOUT = 0.71 206 mA I Low Clamp Current –5 < VIN ≤ –1 –25 + (VIN + 1)/0.015 mA CL 4 slew Output Rise Slew Rate 0.4 V to 2.4 V load 15V/ns R 4 slew Output Fall Slew Rate 2.4 V to 0.4 V load 15V/ns F Notes: 1. Refer to the V/I curves in Figure 2-7 on page 2-22. Switching current characteristics for REQ# and GNT# are permitted to be one half of that specified here; i.e., half size output drivers may be used on these signals. This specification does not apply to CLK and RST#, which are system outputs. The “Switching Current High” specification is not relevant to SERR#, INTA#, INTB#, INTC#, and INTD#, which are open drain outputs. 2. Note that this segment of the minimum current curve is drawn from the AC drive point directly to the DC drive point rather than toward the voltage rail (as is done in the pull-down curve). This difference is intended to allow for an optional N-channel pull-up. 3. Maximum current requirements must be met as drivers pull beyond the last step voltage. Equations defining these maximums (A and B) are provided with the respective curves in Figure 2-7 on page 2-22. The equation defined maximum should be met by the design. In order to facilitate component testing, a maximum current test point is defined for each side of the output driver. 4. This parameter is to be interpreted as the cumulative edge rate across the specified range, rather than the instantaneous rate at any point within the transition range. The specified load is optional; i.e., the designer may elect to meet this parameter with an unloaded output per revision 2.0 of the PCI Local Bus Specification (Figure 2-8). However, adherence to both the maximum and minimum parameters is now required (the maximum is no longer simply a guideline). Since adherence to the maximum slew rate was not required prior to revision 2.1 of the specification, there may be components in the market that have faster edge rates; therefore, motherboard designers must bear in mind that rise and fall times faster than this specification could occur and should ensure that signal integrity modeling accounts for this. Rise slew rate does not apply to open drain outputs. Pin Output Buffer 50 pF Figure 2-8 • 5 V PCI Output Loading Revision 9 2-23 Detailed Specifications Timing Characteristics Table 2-20 • RTSX32SU 5 V PCI I/O Module = 125°C Worst-Case Military Conditions VCCA = 2.25 V, VCCI = 4.5 V, T J Radiation Level = 0 krad (Si) –1 Speed Std. Speed Parameter Description Min. Max. Min. Max. Units 5 V PCI Output Module Timing t Input Data Pad-to-Y High 0.7 0.9 ns INYH t Input Data Pad-to-Y Low 1.1 1.3 ns INYL t Data-to-Pad Low to High 3.4 4.0 ns DLH t Data-to-Pad High to Low 4.1 4.8 ns DHL t Enable-to-Pad, Z to Low 4.1 4.8 ns ENZL t Enable-to-Pad, Z to High 3.4 4.0 ns ENZH t Enable-to-Pad, Low to Z 3.4 4.0 ns ENLZ t Enable-to-Pad, High to Z 4.1 4.8 ns ENHZ 2 d Delta Delay vs. Load Low to High 0.036 0.046 ns/pF TLH 2 d Delta Delay vs. Load High to Low 0.029 0.038 ns/pF THL Notes: 1. Output delays based on 35 pF loading. 2. To obtain the slew rate, substitute the appropriate Delta value, load capacitance, and the VCCI value into the following equation: Slew Rate [V/ns] = ±(0.1 * VCCI - 0.9 * VCCI)/ (C * d |d |d ) load TLH THL THLS where C is the load capacitance driven by the I/O in pF; load d |d |d is the worst case delta value from the datasheet in ns/pF. TLH THL THLS 2-24 Revision 9 RTSX-SU Radiation-Tolerant FPGAs (UMC) Table 2-21 • RTSX72SU 5 V PCI I/O Module Worst-Case Military Conditions VCCA = 2.25 V, VCCI = 4.5 V, T = 125°C J Radiation Level = 0 krad (Si) –1 Speed Std. Speed Parameter Description Min. Max. Min. Max. Units 5 V PCI Output Module Timing t Input Data Pad-to-Y High 0.7 0.9 ns INYH t Input Data Pad-to-Y Low 1.1 1.3 ns INYL t Data-to-Pad Low to High 3.5 4.1 ns DLH t Data-to-Pad High to Low 4.3 5.1 ns DHL t Enable-to-Pad, Z to Low 4.3 5.1 ns ENZL t Enable-to-Pad, Z to High 3.5 4.1 ns ENZH t Enable-to-Pad, Low to Z 3.5 4.1 ns ENLZ t Enable-to-Pad, High to Z 4.3 5.1 ns ENHZ 2 d Delta Delay vs. Load Low to High 0.036 0.046 ns/pF TLH 2 d Delta Delay vs. Load High to Low 0.029 0.038 ns/pF THL Notes: 1. Output delays based on 35 pF loading. 2. To obtain the slew rate, substitute the appropriate Delta value, load capacitance, and the VCCI value into the following equation: Slew Rate [V/ns] = ±(0.1 * VCCI - 0.9 * VCCI)/ (C * d |d |d ) load TLH THL THLS where C is the load capacitance driven by the I/O in pF; load d |d |d is the worst case delta value from the datasheet in ns/pF. TLH THL THLS Revision 9 2-25 Detailed Specifications 3.3 V PCI The RTSX-SU family supports 3.3 V PCI and is compliant with the PCI Local Bus Specification Rev. 2.1. Table 2-22 • 3.3 V PCI DC Specifications Symbol Parameter Condition Min. Max. Units VCCA Supply Voltage for Array 2.25 2.75 V VCCI Supply Voltage for I/Os 3.0 3.6 V VIH Input High Voltage 0.5 VCCI VCCI + 0.5 V VIL Input Low Voltage –0.5 0.3 VCCI V 1 I Input Pull-up Voltage 0.7 VCCI V IPU 2 I /I Input Leakage Current 0 < VIN < VCCI ±20 µA IL IH VOH Output High Voltage I = –500 µA 0.9 VCCI V OUT VOL Output Low Voltage I = 1500 µA 0.1 VCCI V OUT 3 C Input Pin Capacitance 10 pF IN C CLK Pin Capacitance 5 12 pF CLK VMEAS Trip point for Input buffers 0.4 * VCCI V Output buffer measuring point - rising edge 0.285 * VCCI Output buffer measuring point - falling edge 0.615 * VCCI Notes: 1. This specification should be guaranteed by design. It is the minimum voltage to which pull-up resistors are calculated to pull a floated network. Applications sensitive to static power utilization should assure that the input buffer is conducting minimum current at this input VIN. 2. Input leakage currents include hi-Z output leakage for all bidirectional buffers with tristate outputs. 3. Absolute maximum pin capacitance for a PCI input is 10 pF (except for CLK) with an exception granted to motherboard- only devices, which could be up to 16 pF, in order to accommodate PGA packaging. This means that components for expansion boards would need to use alternatives to ceramic PGA packaging. 4. For AC signals, the input signal may undershoot during transitions to –1.2 V for no longer than 11 ns. Current during the transition must not exceed 95 mA. 5. For AC signals, the input signal may overshoot during transitions to VCCI + 1.2 V for no longer than 11 ns. Current during the transition must not exceed 95 mA. 150.0 I Max. Specification OL I OL 100.0 50.0 I Min. Specification OL 0.0 0 0.5 1 1.5 2 2.5 3 3.5 4 –50.0 I Min. Specification OH I Max. Specification I OH OH –100.0 –150.0 Voltage Out (V) Figure 2-9 • 3.3 V PCI V/I Curve for the RTSX-SU Family 2-26 Revision 9 Current (mA) RTSX-SU Radiation-Tolerant FPGAs (UMC) Equation C I = (98.0/VCCI) * (VOUT – VCCI) * (VOUT + 0.4 VCCI) OH for VCCI > VOUT > 0.7 VCCI Equation D I = (256/VCCI) * VOUT * (VCCI – VOUT) OL for 0 V < VOUT < 0.18 VCCI Table 2-23 • 3.3 V PCI AC Specifications Symbol Parameter Condition Min. Max. Units 1 Switching Current 0 < VOUT ≤ 0.3 VCCI –12 VCCI mA I OH(AC) High 1 0.3 VCCI ≤ VOUT < 0.9 VCCI (–17.1 + (VCCI – VOUT)) mA 1, 2 0.7 VCCI < VOUT < VCCI "Equation C" 2 (Test Point) VOUT = 0.7 VCC –32 VCCI mA 1 I Switching Current VCCI > VOUT ≥ 0.6 VCCI 16 VCCI mA OL(AC) Low 1 0.6 VCCI > VOUT > 0.1 VCCI (26.7 VOUT mA ) 1, 2 0.18 VCCI > VOUT > 0 "Equation D" 2 (Test Point) VOUT = 0.18 VCC 38 VCCI mA I Low Clamp Current –3 < VIN ≤ –1 –25 + (VIN + 1)/0.015 mA CL I High Clamp Current VCCI + 4 > VIN ≥ VCCI + 1 25 + (VIN – VCCI – 1)/0.015 mA CH 3 slew Output Rise Slew 0.2 VCCI to 0.6 VCCI load 14V/ns R Rate 3 slew Output Fall Slew 0.6 VCCI to 0.2 VCCI load 14V/ns F Rate Notes: 1. Refer to the V/I curves in Figure 2-9 on page 2-26. Switching current characteristics for REQ# and GNT# are permitted to be one half of that specified here; i.e., half-size output drivers may be used on these signals. This specification does not apply to CLK and RST#, which are system outputs. The “Switching Current High” specification is not relevant to SERR#, INTA#, INTB#, INTC#, and INTD#, which are open drain outputs. 2. Maximum current requirements must be met as drivers pull beyond the last step voltage. Equations defining these maximums (C and D) are provided with the respective curves in Figure 2-9 on page 2-26. The equation defined maximum should be met by the design. In order to facilitate component testing, a maximum current test point is defined for each side of the output driver. 3. This parameter is to be interpreted as the cumulative edge rate across the specified range, rather than the instantaneous rate at any point within the transition range. The specified load is optional (Figure 2-10); i.e., the designer may elect to meet this parameter with an unloaded output per the latest revision of the PCI Local Bus Specification. However, adherence to both maximum and minimum parameters is required (the maximum is no longer simply a guideline). Rise slew rate does not apply to open drain outputs. Pin 1/2 in. max. Pin 1/2 in. max. Output Output Buffer 10 pF Buffer VCC 1 k/25 Ω 1 k/25 Ω 10 pF Figure 2-10 • 3.3 V PCI Output Loading Revision 9 2-27 Detailed Specifications Timing Characteristics Table 2-24 • RTSX32SU 3.3 V PCI I/O Module = 125°C Worst-Case Military Conditions VCCA = 2.25 V, VCCI = 3.0 V, T J Radiation Level = 0 krad (Si) –1 Speed Std.’Speed Parameter Description Min. Max. Min. Max. Units 3.3 V PCI Output Module Timing t Input Data Pad-to-Y High 0.8 0.9 ns INYH t Input Data Pad-to-Y Low 0.9 1.1 ns INYL t Data-to-Pad Low to High 3.0 3.5 ns DLH t Data-to-Pad High to Low 3.0 3.5 ns DHL t Enable-to-Pad, Z to Low 3.0 3.5 ns ENZL t Enable-to-Pad, Z to High 3.0 3.5 ns ENZH t Enable-to-Pad, Low to Z 3.0 3.5 ns ENLZ t Enable-to-Pad, High to Z 3.0 3.5 ns ENHZ 2 d Delta Delay vs. Load Low to High 0.067 0.085 ns/pF TLH 2 d Delta Delay vs. Load High to Low 0.031 0.040 ns/pF THL Notes: 1. Output delays based on 35 pF loading. 2. To obtain the slew rate, substitute the appropriate Delta value, load capacitance, and the VCCI value into the following equation: Slew Rate [V/ns] = ±(0.1 * VCCI - 0.9 * VCCI)/ (C * d |d |d ) load TLH THL THLS where C is the load capacitance driven by the I/O in pF; load d |d |d is the worst case delta value from the datasheet in ns/pF. TLH THL THLS 2-28 Revision 9 RTSX-SU Radiation-Tolerant FPGAs (UMC) Table 2-25 • RTSX72SU 3.3 V PCI I/O Module Worst-Case Military Conditions VCCA = 2.25 V, VCCI = 3.0 V, T = 125°C J Radiation Level = 0 krad (Si) –1 Speed Std. Speed Parameter Description Min. Max. Min. Max. Units 3.3 V PCI Output Module Timing t Input Data Pad-to-Y High 0.7 0.8 ns INYH t Input Data Pad-to-Y Low 0.9 1.1 ns INYL t Data-to-Pad Low to High 2.8 3.3 ns DLH t Data-to-Pad High to Low 2.8 3.3 ns DHL t Enable-to-Pad, Z to Low 2.8 3.3 ns ENZL t Enable-to-Pad, Z to High 2.8 3.3 ns ENZH t Enable-to-Pad, Low to Z 2.8 3.3 ns ENLZ t Enable-to-Pad, High to Z 2.8 3.3 ns ENHZ 2 d Delta Delay vs. Load Low to High 0.067 0.085 ns/pF TLH 2 d Delta Delay vs. Load High to Low 0.031 0.040 ns/pF THL Notes: 1. Output delays based on 35 pF loading. 2. To obtain the slew rate, substitute the appropriate Delta value, load capacitance, and the VCCI value into the following equation: Slew Rate [V/ns] = ±(0.1 * V - 0.9 * V )/ (C * d |d |d ) CCI CCI load TLH THL THLS where C is the load capacitance driven by the I/O in pF; load d |d |d is the worst case delta value from the datasheet in ns/pF. TLH THL THLS Revision 9 2-29 Detailed Specifications Module Specifications C-Cell Introduction The C-cell is one of the two logic module types in the RTSX-SU architecture. It is the combinatorial logic resource in the device. The RTSX-SU architecture uses the same C-cell configuration as found in the SX and SX-A families. The C-cell features the following (Figure 2-11): • Eight-input MUX (data: D0-D3, select: A0, A1, B0, B1). User signals can be routed to any one of these inputs. C-cell inputs (A0, A1, B0, B1) can be tied to one of the either the routed or quad clocks (CLKA/B or QCLKA/B/C/D). • Inverter (DB input) can be used to drive a complement signal of any of the inputs to the C-cell. • A hardwired connection (direct connect) to the associated R-cell with a signal propagation time of less than 0.1 ns. This layout of the C-cell enables the implementation of over 4,000 functions of up to five bits. For example, two C-cells can be used together to implement a four-input XOR function in a single cell delay. The C-cell configuration is handled automatically for the user with Microsemi's extensive macro library (refer to the Antifuse Macro Library Guide for a complete listing of available RTSX-SU macros). D0 D1 Y D2 D3 Sa Sb DB A0 B0 A1 B1 Figure 2-11 • C-Cell VCC GND S, A or B 50% 50% VCC S Y 50% 50% A Y GND B t t PD PD VCC Y GND 50% 50% t t PD PD Figure 2-12 • C-Cell Timing Model and Waveforms 2-30 Revision 9 RTSX-SU Radiation-Tolerant FPGAs (UMC) Timing Characteristics Table 2-26 • C-Cell Worst-Case Military Conditions VCCA = 2.25 V, VCCI = 3.0 V T = 125°C, Radiation Level = 0 krad (Si) J –1’Speed Std. Speed Paramet er Description Min.Max.Min.Max. Units C-cell Propagation Delays t Internal Array Module 1.2 1.4 ns PD Note: For dual-module macros, use t + t + t , t + t + t or t + t + PD RD1 PDn RCO RD1 PDn PD1 RD1 t , whichever is appropriate. SUD R-Cell Introduction The R-cell, the sequential logic resource of RTSX-SU devices, is the second logic module type in the RTSX-SU family architecture. The RTSX-SU R-cell is an SEU-enhanced version of the SX and SX-A R- cell (Figure 2-13). The main features of the R-cell include the following: • Direct connection to the adjacent C-cell through the hardwired connection DCIN. DCIN is driven by the DCOUT of an adjacent C-cell via the Direct-Connect routing resource, providing a connection with less than 0.1 ns of routing delay. • The R-cell can be used as a standalone flip-flop. It can be driven by any other C-cell or I/O modules through the regular routing structure (using DIN as a routable data input). This gives the option of using it as a 2:1 MUXed flip-flop as well. • Independent active-low asynchronous clear (CLRB). • Independent active-low asynchronous preset (PSETB). If both CLRB and PSETB are Low, CLRB has higher priority. • Clock can be driven by any of the following (CKP input selects clock polarity): – The high-performance, hardwired, fast clock (HCLK) – One of the two routed clocks (CLKA/B) – One of the four quad clocks (QCLKA/B/C/D) in the case of the RTSX72SU – User signals • S0, S1, PSETB, and CLRB can be driven by CLKA/B, QCLKA/B/C/D (for the RTSX72SU) or user signals. • Routed Data Input and S1 can be driven by user signals. Revision 9 2-31 Detailed Specifications As with the C-cell, the configuration of the R-cell to perform various functions is handled automatically for the user through Microsemi's extensive macro library (see the Antifuse Macro Library Guide for a complete listing of available RTSX-SU macros). Routed S1 Data Input S0 PSETB Direct Connect DQ Y Input HCLK CLKA, CLRB CLKB, Internal Logic CKS CKP Figure 2-13 • R-Cell SEU-Hardened D Flip-Flop 2 In order to meet the stringent SEU requirements of a LET threshold greater than 40MeV-cm /gm, the internal design of the R-cell was modified without changing the functionality of the cell. Figure 2-14 is a simplified representation of how the D flip-flop in the R-cell is implemented in the SX-A architecture. The flip-flop consists of a master and a slave latch gated by opposite edges of the clock. Each latch is constructed by feeding back the output to the input stage. The potential problem in a space environment is that either of the latches can change state when hit by a particle with enough energy. To achieve the SEU requirements, the D flip-flop in the RTSX-SU R-cell is enhanced (Figure 2-15). Both the master and slave latches are each implemented with three latches. The asynchronous self-correcting feedback paths of each of the three latches is voted with the outputs of the other two latches. If one of the three latches is struck by an ion and starts to change state, the voting with the other two latches prevents the change from feeding back and permanently latching. Care was taken in the layout to ensure that a single ion strike could not affect more than one latch. Figure 2-16 shows a simplified schematic of the test circuitry that has been added to test the functionality of all the components of the flip-flop. The inputs to each of the three latches are independently controllable so the voting circuitry in the asynchronous self-correcting feedback paths can be tested exhaustively. This testing is performed on an unprogrammed array during wafer sort, final test, and post-burn-in test. This test circuitry cannot be used to test the flip-flops once the device has been programmed. D Q CLK CLK Figure 2-14 • SX-A R-Cell Implementation of a D Flip-Flop 2-32 Revision 9 RTSX-SU Radiation-Tolerant FPGAs (UMC) D Q CLK CLK Voter Gate CLK CLK CLK CLK CLK CLK Figure 2-15 • RTSX-SU R-Cell Implementation of D Flip-Flop Using Voter Gate Logic D Q Tst1 Voter Gate Tst2 Tst3 CLK Test Circuitry Figure 2-16 • R-Cell Implementation – Test Circuitry Revision 9 2-33 Detailed Specifications PRE D Q CLK CLR (Positive edge triggered) t HD D t HPWH t t t SUD HP RPWH CLK t t RCO HPWL t RPWL Q t PRESET t CLR CLR t WASYN PRESET Figure 2-17 • R-Cell Timing Models and Waveforms Timing Characteristics Table 2-27 • R-Cell Worst-Case Military Conditions VCCA = 2.25 V, VCCI = 3.0 V, T = 125°C J Radiation Level = 0 krad (Si) –1 Speed Std. Speed Parameter Description Min. Max. Min. Max. Units R-Cell Propagation Delays t Sequential Clock-to-Q 1.0 1.2 ns RCO t Asynchronous Clear-to-Q 0.8 1.0 ns CLR t Asynchronous Preset-to-Q 1.1 1.3 ns PRESET t Flip-Flop Data Input Set-Up 0.8 1.0 ns SUD t Flip-Flop Data Input Hold 0.0 0.0 ns HD t Asynchronous Pulse Width 2.8 3.3 ns WASYN t Asynchronous Recovery Time 0.7 0.8 ns RECASYN t Asynchronous Hold Time 0.7 0.8 ns HASYN 2-34 Revision 9 RTSX-SU Radiation-Tolerant FPGAs (UMC) Routing Specifications Routing Resources The routing structure found in RTSX-SU devices enables any logic module to be connected to any other logic module in the device while retaining high performance. There are multiple paths and routing resources that can be used to route one logic module to another, both within a SuperCluster and elsewhere on the chip. There are three primary types of routing within the RTSX-SU architecture: DirectConnect, FastConnect, and Vertical and Horizontal Routing. DirectConnect DirectConnects provide a high-speed connection between an R-cell and its adjacent C-cell (Figure 1-3 and Figure 1-4 on page 1-5). This connection can be made from the Y output of the C-cell to the DirectConnect input of the R-cell by configuring of the S0 line of the R-cell. This provides a connection that does not require an antifuse and has a delay of less than 0.1 ns. FastConnect For high-speed routing of logic signals, FastConnects can be used to build a short distance connection using a single antifuse (Figure 1-3 and Figure 1-4 on page 1-5). FastConnects provide a maximum delay of 0.4 ns. The outputs of each logic module connect directly to the output tracks within a SuperCluster. Signals on the output tracks can then be routed through a single antifuse connection to drive the inputs of logic modules either within one SuperCluster or in the SuperCluster immediately below. Horizontal and Vertical Routing In addition to DirectConnect and FastConnect, the architecture makes use of two globally-oriented routing resources known as segmented routing and high-drive routing. Microsemi’s segmented routing structure provides a variety of track lengths for extremely fast routing between SuperClusters. The exact combination of track lengths and antifuses within each path is chosen by the 100-percent-automatic place-and-route software to minimize signal propagation delays. Critical Nets and Typical Nets Propagation delays are expressed only for typical nets, which are used for the initial design performance evaluation. Critical net delays can then be applied to the most time-critical paths. Critical nets are determined by net property assignment prior to placement and routing. Up to six percent of the nets in a design may be designated as critical, while 90 percent of the nets in a design are typical. Long Tracks Some nets in the design use long tracks. Long tracks are special routing resources that span multiple rows, columns, or modules. Long tracks employ three and sometimes five antifuse connections. This increases capacitance and resistance results in longer net delays for macros connected to long tracks. Typically up to six percent of nets in a fully utilized device require long tracks. Long tracks can cause a delay from 4.0 ns to 8.4 ns. This additional delay is represented statistically in higher fanout routing delays in the "Timing Characteristics" section on page 2-36. Revision 9 2-35 Detailed Specifications Timing Characteristics Table 2-28 • RTSX32SU Worst-Case Military Conditions VCCA = 2.25 V, VCCI = 3.0 V, T = 125°C J Radiation Level = 0 krad (Si) –1 Speed Std. Speed Parameter Description Min. Max. Min. Max. Units Predicted Routing Delays t FO = 1 Routing Delay, DirectConnect 0.1 0.1 ns DC t FO = 1 Routing Delay, FastConnect 0.4 0.4 ns FC t FO = 1 Routing Delay 0.8 0.9 ns RD1 t FO = 2 Routing Delay 1.0 1.2 ns RD2 t FO = 3 Routing Delay 1.4 1.6 ns RD3 t FO = 4 Routing Delay 1.5 1.8 ns RD4 t FO = 8 Routing Delay 2.9 3.4 ns RD8 t FO = 12 Routing Delay 4.0 4.7 ns RD12 Note: Routing delays are for typical designs across worst-case operating conditions. These parameters should be used for estimating device performance. Post-route timing analysis or simulation is required to determine actual worst-case performance. Table 2-29 • RTSX72SU Worst-Case Military Conditions VCCA = 2.25 V, VCCI = 3.0 V, T = 125°C J Radiation Level = 0 krad (Si) –1 Speed Std. Speed Parameter Description Min. Max. Min. Max. Units Predicted Routing Delays t FO = 1 Routing Delay, DirectConnect 0.1 0.1 ns DC t FO = 1 Routing Delay, FastConnect 0.4 0.4 ns FC t FO = 1 Routing Delay 0.9 1.0 ns RD1 t FO = 2 Routing Delay 1.2 1.4 ns RD2 t FO = 3 Routing Delay 1.8 2.0 ns RD3 t FO = 4 Routing Delay 1.9 2.3 ns RD4 t FO = 8 Routing Delay 3.7 4.3 ns RD8 t FO = 12 Routing Delay 5.1 6.0 ns RD12 Note: Routing delays are for typical designs across worst-case operating conditions. These parameters should be used for estimating device performance. Post-route timing analysis or simulation is required to determine actual worst-case performance. 2-36 Revision 9 RTSX-SU Radiation-Tolerant FPGAs (UMC) Global Resources One of the most important aspects of any FPGA architecture is its global resource or clock structure. The RTSX-SU family provides flexible and easy-to-use global resources without the limitations normally found in other FPGA architectures. The RTSX-SU architecture contains three types of global resources, the HCLK (hardwired clock) and CLK (routed clock) and in the RTSX72SU, QCLK (quadrant clock). Each RTSX-SU device is provided with one HCLK and two CLKs. The RTSX72SU has an additional four QCLKs. Hardwired Clock The hardwired (HCLK) is a low-skew network that can directly drive the clock inputs of all R-cells in the device with no antifuse in the path. The HCLK is available everywhere on the chip. Upon power-up of the RTSX-SU device, four clock pulses must be detected on HCLK before the clock signal will be propagated to registers in the device. Routed Clocks The routed clocks (CLKA and CLKB) are low-skew networks that can drive the clock inputs of all R-cells in the device (logically equivalent to the HCLK). CLK has the added flexibility in that it can drive the S0 (Enable), S1, PSETB, and CLRB inputs of R-cells as well as any of the inputs of any C-cell in the device. This allows CLKs to be used not only as clocks but also for other global signals or high fanout nets. Both CLKs are available everywhere on the chip. If CLKA or CLKB pins are not used or sourced from signals, then these pins must be set as Low or High on the board. They must not be left floating (except in RTSX72SU, where these clocks can be configured as regular I/Os). Revision 9 2-37 Detailed Specifications Quadrant Clocks The RTSX72SU device provides four quadrant clocks (QCLKA, QCLKB, QCLKC, QCLKD) to the user, which can be sourced from external pins or from internal logic signals within the device. Each of these clocks can individually drive up to one full quadrant of the chip, or they can be grouped together to drive multiple quadrants (Figure 2-18). If QCLKs are not used as quadrant clocks, they can behave as regular I/Os. See Microsemi’s application note Using A54SX72A and RT54SX72S Quadrant Clocks for more information. 4 QCLKBUFS 4 Quadrant 2 Quadrant 3 5:1 5:1 QCLKINT QCLKINT (to array) (to array) 4 Quadrant 0 Quadrant 1 5:1 5:1 QCLKINT QCLKINT (to array) (to array) Figure 2-18 • RTSX-SU QCLK Structure 2-38 Revision 9 RTSX-SU Radiation-Tolerant FPGAs (UMC) Timing Characteristics Table 2-30 • RTSX32SU at VCCI = 3.0 V = 125°C Worst-Case Military Conditions VCCA = 2.25 V, VCCI = 3.0 V, T J Radiation Level = 0 krad (Si) –1 Speed Std. Speed Parameter Description Min. Max. Min. Max. Units Dedicated (Hardwired) Array Clock Network t Pad to R-Cell Input Low to High 3.9 4.6 ns HCKH t Pad to R-Cell Input High to Low 3.9 4.6 ns HCKL t Minimum Pulse Width High 2.1 2.5 ns HPWH t Minimum Pulse Width Low 2.1 2.5 ns HPWL t Maximum Skew 1.6 1.9 ns HCKSW t Minimum Period 4.2 5.0 ns HP f Maximum Frequency 238 200 MHz HMAX Routed Array Clock Networks t Pad to R-cell Input High to Low (Light Load)) 4.2 4.9 ns RCKH t Pad to R-cell Input Low to High (Light Load)) 3.9 4.6 ns RCHKL t Pad to R-cell Input Low to High (50% Load) 5.0 5.9 ns RCKH t Pad to R-cell Input High to Low (50% Load) 4.3 5.1 ns RCKL t Pad to R-cell Input Low to High (100% Load) 5.6 6.5 ns RCKH t Pad to R-cell Input High to Low (100% Load) 4.9 5.7 ns RCKL t Minimum Pulse Width High 2.1 2.5 ns RPWH t Minimum Pulse Width Low 2.1 2.5 ns RPWL t Maximum Skew (Light Load) 2.8 3.3 ns RCKSW t Maximum Skew (50% Load) 2.8 3.3 ns RCKSW t Maximum Skew (100% Load) 2.8 3.3 ns RCKSW t Minimum Period 4.2 5.0 ns RP f Maximum Frequency 238 200 MHz RMAX Revision 9 2-39 Detailed Specifications Table 2-31 • RTSX32SU at VCCI = 4.5 V Worst-Case Military Conditions VCCA = 2.25 V, VCCI = 4.5 V, T = 125°C J Radiation Level = 0 krad (Si) –1 Speed Std. Speed Parameter Description Min. Max. Min. Max. Units Dedicated (Hardwired) Array Clock Network t Pad to R-Cell Input Low to High 3.9 4.6 ns HCKH t Pad to R-Cell Input High to Low 3.9 4.6 ns HCKL t Minimum Pulse Width High 2.1 2.5 ns HPWH t Minimum Pulse Width Low 2.1 2.5 ns HPWL t Maximum Skew 1.6 1.9 ns HCKSW t Minimum Period 4.2 5.0 ns HP f Maximum Frequency 238 200 MHz HMAX Routed Array Clock Networks t Pad to R-cell Input High to Low (Light Load)) 3.9 4.6 ns RCKH t Pad to R-cell Input Low to High (Light Load)) 3.7 4.4 ns RCHKL t Pad to R-cell Input Low to High (50% Load) 4.7 5.6 ns RCKH t Pad to R-cell Input High to Low (50% Load) 4.1 4.9 ns RCKL t Pad to R-cell Input Low to High (100% Load) 5.3 6.2 ns RCKH t Pad to R-cell Input High to Low (100% Load) 4.7 5.5 ns RCKL t Minimum Pulse Width High 2.1 2.5 ns RPWH t Minimum Pulse Width Low 2.1 2.5 ns RPWL t Maximum Skew (Light Load) 2.8 3.3 ns RCKSW t Maximum Skew (50% Load) 2.8 3.3 ns RCKSW t Maximum Skew (100% Load) 2.8 3.3 ns RCKSW t Minimum Period 4.2 5.0 ns RP f Maximum Frequency 238 200 MHz RMAX 2-40 Revision 9 RTSX-SU Radiation-Tolerant FPGAs (UMC) Table 2-32 • RTSX72SU at VCCI = 3.0 V Worst-Case Military Conditions VCCA = 2.25 V, VCCI = 3.0 V, T = 125°C J Radiation Level = 0 krad (Si) –1 Speed Std. Speed Parameter Description Min. Max. Min. Max. Units Dedicated (Hardwired) Array Clock Network t Pad to R-cell Input Low to High 3.2 3.8 ns HCKH t Pad to R-cell Input High to Low 3.5 4.1 ns HCKL t Minimum Pulse Width High 2.7 3.2 ns HPWH t Minimum Pulse Width Low 2.7 3.2 ns HPWL t Maximum Skew 2.7 3.1 ns HCKSW t Minimum Period 5.4 6.4 ns HP f Maximum Frequency 185 156 MHz HMAX Routed Array Clock Networks t Pad to R-cell Input Low to High (Light Load)) 5.7 6.7 ns RCKH t Pad to R-cell Input High to Low (Light Load) 6.5 7.7 ns RCKL t Pad to R-cell Input Low to High (50% Load) 5.7 6.7 ns RCKH t Pad to R-cell Input High to Low (50% Load) 6.5 7.7 ns RCKL t Pad to R-cell Input Low to High (100% Load) 5.7 6.7 ns RCKH t Pad to R-cell Input High to Low (100% Load) 6.5 7.7 ns RCKL t Minimum Pulse Width High 2.7 3.2 ns RPWH t Minimum Pulse Width Low 2.7 3.2 ns RPWL t Maximum Skew (Light Load) 5.1 6.0 ns RCKSW t Maximum Skew (50% Load) 4.9 5.8 ns RCKSW t Maximum Skew (100% Load) 4.9 5.8 ns RCKSW t Minimum Period 5.4 6.4 ns RP f Maximum Frequency 185 156 MHz RMAX Quadrant Array Clock Networks t Pad to R-cell Input Low to High (Light Load) 3.6 4.2 ns QCKH t Pad to R-cell Input High to Low (Light Load) 3.6 4.2 ns QCKL t Pad to R-cell Input Low to High (50% Load) 3.7 4.3 ns QCKH t Pad to R-cell Input High to Low (50% Load) 3.9 4.5 ns QCKL t Pad to R-cell Input Low to High (100% Load) 4.0 4.7 ns QCKH t Pad to R-cell Input High to Low (100% Load) 4.1 4.8 ns QCKL t Minimum Pulse Width High 2.7 3.2 ns QPWH t Minimum Pulse Width Low 2.7 3.2 ns QPWL t Maximum Skew (Light Load) 0.6 0.7 ns QCKSW t Maximum Skew (50% Load) 1.0 1.1 ns QCKSW t Maximum Skew (100% Load) 1.0 1.1 ns QCKSW t Minimum Period 5.4 6.4 ns QP f Maximum Frequency 185 156 MHz QMAX Revision 9 2-41 Detailed Specifications Table 2-33 • RTSX72SU at VCCI = 4.5 V Worst-Case Military Conditions VCCA = 2.25 V, VCCI = 4.5 V, T = 125°C J Radiation Level = 0 krad (Si) –1 Speed Std. Speed Parameter Description Min. Max. Min. Max. Units Dedicated (Hardwired) Array Clock Network t Pad to R-cell Input Low to High 4.1 4.8 ns HCKH t Pad to R-cell Input High to Low 4.1 4.8 ns HCKL t Minimum Pulse Width High 2.8 3.3 ns HPWH t Minimum Pulse Width Low 2.8 3.3 ns HPWL t Maximum Skew 3.2 3.7 ns HCKSW t Minimum Period 5.6 6.6 ns HP f Maximum Frequency 179 152 MHz HMAX Routed Array Clock Networks t Pad to R-cell Input Low to High (Light Load)) 6.8 8.0 ns RCKH t Pad to R-cell Input High to Low (Light Load) 8.2 9.7 ns RCKL t Pad to R-cell Input Low to High (50% Load) 6.8 8.0 ns RCKH t Pad to R-cell Input High to Low (50% Load) 8.2 9.7 ns RCKL t Pad to R-cell Input Low to High (100% Load) 6.8 8.0 ns RCKH t Pad to R-cell Input High to Low (100% Load) 8.2 9.7 ns RCKL t Minimum Pulse Width High 2.8 3.3 ns RPWH t Minimum Pulse Width Low 2.8 3.3 ns RPWL t Maximum Skew (Light Load) 7.0 8.2 ns RCKSW t Maximum Skew (50% Load) 6.8 8.0 ns RCKSW t Maximum Skew (100% Load) 6.8 8.0 ns RCKSW t Minimum Period 5.6 6.6 ns QP f Maximum Frequency 179 152 MHz QMAX Quadrant Array Clock Networks t Pad to R-cell Input Low to High (Light Load)) 3.9 4.6 ns QCKH t Pad to R-cell Input High to Low (Light Load) 4.2 4.9 ns QCKL t Pad to R-cell Input Low to High (50% Load) 4.2 4.9 ns QCKH t Pad to R-cell Input High to Low (50% Load) 4.5 5.3 ns QCKL t Pad to R-cell Input Low to High (100% Load) 4.5 5.3 ns QCKH t Pad to R-cell Input High to Low (100% Load) 5.0 5.9 ns QCKL t Minimum Pulse Width High 2.8 3.3 ns QPWH t Minimum Pulse Width Low 2.8 3.3 ns QPWL t Maximum Skew (Light Load) 0.7 0.8 ns QCKSW t Maximum Skew (50% Load) 1.3 1.5 ns QCKSW t Maximum Skew (100% Load) 1.4 1.6 ns QCKSW t Minimum Period 5.6 6.6 ns QP f Maximum Frequency 179 152 MHz QMAX 2-42 Revision 9 RTSX-SU Radiation-Tolerant FPGAs (UMC) Global Resource Access Macros The user can configure which global resource is used in the design as well as how each global resource is driven through the use of the following macros: • HCLKBUF – used to drive the hardwired clock (HCLK) in both devices from an external pin • CLKBUF and CLKBUFI – noninverting and inverting inputs used to drive either routed clock (CLKA or CLKB) in both devices from external pins • CLKINT and CLKINTI – noninverting and inverting inputs used to drive either routed clock (CLKA or CLKB) in both devices from internal logic • QCLKBUF and QCLKBUFI – noninverting and inverting inputs used to drive quadrant routed clocks (QCLKA/B/C/D) in the RTSX72SU from external pins • QCLKINT and QCLKINTI – noninverting and inverting inputs used to drive quadrant routed clocks (QCLKA/B/C/D) in the RTSX72SU from internal logic • QCLKBIBUF and QCLUKBIBUFI – noninverting and inverting inputs used to drive quadrant routed clocks (QCLKA/B/C/D) in the RTSX72SU alternatively from either external pins or internal logic Figure 2-19, Figure 2-20, and Figure 2-21 illustrate the various global-resource access macros. Constant Load Clock Network HCLKBUF Figure 2-19 • Hardwired Clock Buffer Clock Network From Internal Logic CLKBUF CLKBUFI CLKINT CLKINTI Figure 2-20 • Routed Clock Buffers in RTSX32SU Revision 9 2-43 Detailed Specifications OE From Internal Logic Clock Network From Internal Logic CLKBUF QCLKBUF CLKBUFI QCLKBUFI CLKINT QCLKINT CLKINTI QCLKINTI CLKBIBUF QCLKBIBUF CLKBIBUFI QCLKBIBUFI Figure 2-21 • Routed and Quadrant Clock Buffers in RTSX72SU 2-44 Revision 9 RTSX-SU Radiation-Tolerant FPGAs (UMC) Other Architectural Features JTAG Interface All RTSX-SU devices are IEEE 1149.1 compliant and offer superior diagnostic and testing capabilities by providing Boundary Scan Testing (BST) and probing capabilities. The BST function is controlled through special JTAG pins (TMS, TDI, TCK, TDO, and TRST). The functionality of the JTAG pins is defined by two available modes: dedicated and flexible (Table 2-34). Note that TRST and TMS cannot be employed as user I/Os in either mode. Table 2-34 • Boundary Scan Pin Functionality Dedicated Test Mode Flexible Mode TCK, TDI, TDO are dedicated BST pins TCK, TDI, TDO are flexible and may be used as user I/Os No need for pull-up resistor for TMS Use a pull-up resistor of 10 kΩ on TMS Dedicated Mode In dedicated mode, all JTAG pins are reserved for BST; users cannot employ them as regular I/Os. An 3 ) is automatically enabled on both TMS and TDI internal pull-up resistor (on the order of 17 kΩ to 22 kΩ pins, and the TMS pin will function as defined in the IEEE 1149.1 (JTAG) specification. To enter dedicated mode, users need to reserve the JTAG pins in Microsemi’s Designer software during device selection. To reserve the JTAG pins, users can check the Reserve JTAG box in the Device Selection Wizard in the Designer software (Figure 2-22). Figure 2-22 • Device Selection Wizard Flexible Mode In flexible mode, TDI, TCK, and TDO may be employed as either user I/Os or as JTAG input pins. The internal resistors on the TMS and TDI pins are not present in flexible JTAG mode. To enter the flexible mode, users need to clear the Reserve JTAG box in the Device Selection Wizard in Designer software. TDI, TCK, and TDO pins may function as user I/Os or BST pins in flexible mode. This functionality is controlled by the BST TAP controller. The TAP controller receives two control inputs: TMS and TCK. Upon power-up, the TAP controller enters the Test-Logic-Reset state. In this state, TDI, TCK, and TDO function as user I/Os. The TDI, TCK, and TDO are transformed from user I/Os into BST pins when a rising edge on TCK is detected while TMS is at logic Low. To return to the Test-Logic-Reset state, in the absences of TRST assertion, TMS must be held High for at least five TCK cycles. An external, 10 kΩ pull-up resistor tied to VCCI should be placed on the TMS pin to pull it High by default. Table 2-35 describes the different configurations of the BST pins and their functionality in different modes. Table 2-35 • JTAG Pin Configurations and Functions Mode Designer Reserve JTAG Selection TAP Controller State Dedicated (JTAG) Checked Any Flexible (User I/O) Unchecked Test-Logic-Reset Flexible (JTAG) Unchecked Other 3. On a given device, the value of the internal pull-up resistor varies within 1 kW between the TMS and TDI pins. Revision 9 2-45 Detailed Specifications TRST Pin The TRST pin functions as a dedicated boundary scan reset pin. An internal pull-up resistor is permanently enabled on the TRST pin. Additionally, the TRST pin must be grounded for flight applications. This will prevent single event upsets (SEU) in the TAP controller from inadvertently placing the device into JTAG mode. Probing Capabilities RTSX-SU devices also provide internal probing capability that is accessed with the JTAG pins. Silicon Explorer II Probe Interface Silicon Explorer II is an integrated hardware and software solution that, in conjunction with Designer software, allows users to examine any of the internal nets of the device while it is operating in a prototype or a production system. The user can probe two nodes at a time without changing the placement or routing of the design and without using any additional device resources. Highlighted nets in Designer’s ChipEditor can be accessed using Silicon Explorer II in order to observe their real time values. Silicon Explorer II's noninvasive method does not alter timing or loading effects, thus shortening the debug cycle. In addition, Silicon Explorer II does not require relayout or additional MUXes to bring signals out to external pins, which is necessary when using programmable logic devices from other suppliers. By eliminating multiple place-and-route cycles, the integrity of the design is maintained throughout the debug process. Both members of the RTSX-SU family have two external pads: PRA and PRB. These can be used to bring out two probe signals from the device. To disallow probing, the SFUS security fuse in the silicon signature has to be programmed. Table 2-36 shows the possible device configuration options and their effects on probing. During probing, the Silicon Explorer II Diagnostic Hardware is used to control the TDI, TCK, TMS, and TDO pins to select the desired nets for debugging. The user simply assigns the selected internal nets in the Silicon Explorer II software to the PRA/PRB output pins for observation. Probing functionality is activated when the BST pins are in JTAG mode and the TRST pin is driven High. If the TRST pin is held Low, the TAP controller will remain in the Test-Logic-Reset state, so no probing can be performed. Silicon Explorer II automatically places the device into JTAG mode, but the user must drive the TRST pin High or allow the internal pull-up resistor to pull TRST High. Silicon Explorer II connects to the host PC using a standard serial port connector. Connections to the circuit board are achieved using a nine-pin D-Sub connector (Figure 1-5 on page 1-8). Once the design has been placed-and-routed and the RTSX-SU device has been programmed, Silicon Explorer II can be connected and the Silicon Explorer software can be launched. Silicon Explorer II comes with an additional optional PC-hosted tool that emulates an 18-channel logic analyzer. Two channels are used to monitor two internal nodes, and 16 channels are available to probe external signals. The software included with the tool provides the user with an intuitive interface that allows for easy viewing and editing of signal waveforms. Table 2-36 • Device Configuration Options for Probe Capability 1 1 JTAG Mode TRST Security Fuse Programmed PRA and PRB TDI, TCK, and TDO 2 Dedicated Low No User I/O Probing Unavailable 2 2 Flexible Low No User I/O User I/O Dedicated High No Probe Circuit Outputs Probe Circuit I/O Flexible High No Probe Circuit Outputs Probe Circuit I/O – – Yes Probe Circuit Secured Probe Circuit Secured Notes: 1. Avoid using the TDI, TCK, TDO, PRA, and PRB pins as input or bidirectional ports during probing. Since these pins are active during probing, input signals will not pass through these pins and may cause contention. 2. If no user signal is assigned to these pins, they will behave as unused I/Os in this mode. Unused pins are automatically tristated by the Designer software. 2-46 Revision 9 RTSX-SU Radiation-Tolerant FPGAs (UMC) Security Fuses Microsemi antifuse FPGAs, with FuseLock technology, offer the highest level of design security available in a programmable logic device. Since antifuse FPGAs are live at power-up, there is no bitstream that can be intercepted, and no bitstream or programming data is ever downloaded to the device, thus making device cloning virtually impossible. In addition, special security fuses are hidden throughout the fabric of the device and may be programmed by the user to thwart attempts to reverse engineer the device by attempting to exploit either the programming or probing interfaces. Both invasive and noninvasive attacks against an RTSX-SU device that access or bypass these security fuses will destroy access to the rest of the device. Refer to the Understanding Actel Antifuse Device Security white paper for more information. Look for this symbol to ensure your valuable IP is protected with the highest level of security available in the industry (Figure 2-23). ® FuseLock Figure 2-23 • FuseLock Logo To ensure maximum security in RTSX-SU devices, it is recommended that the user program the device security fuse (SFUS). When programmed, the Silicon Explorer II testing probes are disabled to prevent internal probing, and the programming interface is also disabled. All JTAG public instructions are still accessible by the user. For more information, refer to the Implementation of Security in Actel Antifuse FPGAs application note. Programming Device programming is supported through the Silicon Sculptor series programmers. These programmers are single-site, robust and compact device-programmers for the PC. Multiple Silicon Sculptor programmers can be daisy-chained and controlled from a single PC host. The number of programmers that can be daisy chained depends on the programmer model. With standalone software for the PC, Silicon Sculptor programmers are designed to allow concurrent programming of multiple units from the same PC when daisy-chained. Silicon Sculptor programmers program devices independently to achieve the fastest programming times possible. Each fuse is verified by Silicon Sculptor programmers to ensure correct programming. Furthermore, at the end of programming, there are integrity tests that are run to ensure that programming was completed properly. Not only do they test programmed and nonprogrammed fuses, Silicon Sculptor programmers also provide a self-test to extensively test their own hardware. Programming an RTSX-SU device using a Silicon Sculptor programmer is similar to programming any other antifuse device. The procedure is as follows: 1. Load the *.AFM file 2. Select the device to be programmed 3. Begin programming When the design is ready to go to production, Microsemi offers volume programming services either through distribution partners or via our In-House Programming Center. For more details on programming RTSX-SU devices, refer to the Silicon Sculptor Software v5.0 for Silicon Sculptor II and Silicon Sculptor 3 Programmer User’s Guide. Find more information on the Microsemi SoC Products Group website: http://www.microsemi.com/soc/products/hardware/program_debug/ss/default.aspx#docs. Revision 9 2-47 3 – Package Pin Assignments CQ84 Pin 1 indicator may be in a different shape for different 84 64 devices. 1 63 21 43 22 42 Note For Package Manufacturing and Environmental information, visit the Resource Center at http://www.microsemi.com/soc/products/solutions/package/default.aspx. Revision 9 3-1 Package Pin Assignments CQ84 CQ84 CQ84 Pin Number RTSX32SU Function Pin Number RTSX32SU Function Pin Number RTSX32SU Function 1I/O 37 GND 73 CLKB 2I/O 38 I/O 74 PRA, I/O 3TMS 39 TDO, I/O 75 I/O 4I/O 40 I/O 76 I/O 5 VCCI 41 I/O 77 I/O 6GND 42 I/O 78 GND 7I/O 43 I/O 79 VCCA 8I/O 44 I/O 80 I/O 9I/O 45 I/O 81 I/O 10 I/O 46 VCCA 82 TCK, I/O 11 TRST 47 VCCI 83 TDI, I/O 12 I/O 48 GND 84 I/O 13 I/O 49 I/O 14 I/O 50 I/O 15 VCCA 51 I/O 16 GND 52 I/O 17 I/O 53 I/O 18 VCCA 54 I/O 19 I/O 55 I/O 20 I/O 56 I/O 21 I/O 57 VCCA 22 I/O 58 GND 23 I/O 59 I/O 24 I/O 60 VCCA 25 I/O 61 GND 26 GND 62 I/O 27 VCCI 63 I/O 28 I/O 64 I/O 29 I/O 65 I/O 30 I/O 66 I/O 31 I/O 67 I/O 32 PRB, I/O 68 VCCI 33 HCLK 69 GND 34 I/O 70 I/O 35 I/O 71 I/O 36 VCCA 72 CLKA 3-2 Revision 9 RTSX-SU Radiation-Tolerant FPGAs (UMC) CQ208 1 156 Pin 1 2 155 3 154 4 153 Ceramic Tie Bar 208-Pin CQFP 49 108 50 107 51 106 52 105 Note For Package Manufacturing and Environmental information, visit the Resource Center at http://www.microsemi.com/soc/products/solutions/package/default.aspx. Revision 9 3-3 158 103 53 208 54 207 55 206 56 205 101 160 159 102 157 104 Package Pin Assignments CQ208 CQ208 RTSX32SU RTSX72SU RTSX32SU RTSX72SU Pin Number Function Function Pin Number Function Function 1GND GND 38 I/O I/O 39 I/O I/O 2 TDI, I/O TDI, I/O 3 I/O I/O 40 VCCI VCCI 4 I/O I/O 41 VCCA VCCA 5 I/O I/O 42 I/O I/O 6 I/O I/O 43 I/O I/O 7 I/O I/O 44 I/O I/O 8 I/O I/O 45 I/O I/O 9 I/O I/O 46 I/O I/O 10 I/O I/O 47 I/O I/O 11 TMS TMS 48 I/O I/O 12 VCCI VCCI 49 I/O I/O 13 I/O I/O 50 I/O I/O 14 I/O I/O 51 I/O I/O 15 I/O I/O 52 GND GND 16 I/O I/O 53 I/O I/O 17 I/O I/O 54 I/O I/O 18 I/O GND 55 I/O I/O 19 I/O VCCA 56 I/O I/O 20 I/O I/O 57 I/O I/O 21 I/O I/O 58 I/O I/O 22 I/O I/O 59 I/O I/O 23 I/O I/O 60 VCCI VCCI 24 I/O I/O 61 I/O I/O 25 NC I/O 62 I/O I/O 26 GND GND 63 I/O I/O 27 VCCA VCCA 64 I/O I/O 28 GND GND 65 NC I/O 29 I/O I/O 66 I/O I/O 30 TRST TRST 67 I/O I/O 31 I/O I/O 68 I/O I/O 32 I/O I/O 69 I/O I/O 33 I/O I/O 70 I/O I/O 34 I/O I/O 71 I/O I/O 35 I/O I/O 72 I/O I/O 36 I/O I/O 73 I/O I/O 37 I/O I/O 74 I/O QCLKA, I/O 3-4 Revision 9 RTSX-SU Radiation-Tolerant FPGAs (UMC) CQ208 CQ208 RTSX32SU RTSX72SU RTSX32SU RTSX72SU Pin Number Function Function Pin Number Function Function 75 I/O I/O 112 I/O I/O 76 PRB, I/O PRB, I/O 113 I/O I/O 77 GND GND 114 VCCA VCCA 78 VCCA VCCA 115 VCCI VCCI 79 GND GND 116 I/O GND 80 NC NC 117 I/O VCCA 81 I/O I/O 118 I/O I/O 82 HCLK HCLK 119 I/O I/O 83 I/O VCCI 120 I/O I/O 84 I/O QCLKB, I/O 121 I/O I/O 85 I/O I/O 122 I/O I/O 86 I/O I/O 123 I/O I/O 87 I/O I/O 124 I/O I/O 88 I/O I/O 125 I/O I/O 89 I/O I/O 126 I/O I/O 90 I/O I/O 127 I/O I/O 91 I/O I/O 128 I/O I/O 92 I/O I/O 129 GND GND 93 I/O I/O 130 VCCA VCCA 94 I/O I/O 131 GND GND 95 I/O I/O 132 NC I/O 96 I/O I/O 133 I/O I/O 97 I/O I/O 134 I/O I/O 98 VCCI VCCI 135 I/O I/O 99 I/O I/O 136 I/O I/O 100 I/O I/O 137 I/O I/O 101 I/O I/O 138 I/O I/O 102 I/O I/O 139 I/O I/O 103 TDO, I/O TDO, I/O 140 I/O I/O 104 I/O I/O 141 I/O I/O 105 GND GND 142 I/O I/O 106 I/O I/O 143 I/O I/O 107 I/O I/O 144 I/O I/O 108 I/O I/O 145 VCCA VCCA 109 I/O I/O 146 GND GND 110 I/O I/O 147 I/O I/O 111 I/O I/O 148 VCCI VCCI Revision 9 3-5 Package Pin Assignments CQ208 CQ208 RTSX32SU RTSX72SU RTSX32SU RTSX72SU Pin Number Function Function Pin Number Function Function 149 I/O I/O 186 PRA, I/O PRA, I/O 150 I/O I/O 187 I/O VCCI 151 I/O I/O 188 I/O I/O 152 I/O I/O 189 I/O I/O 153 I/O I/O 190 I/O QCLKC, I/O 154 I/O I/O 191 I/O I/O 155 I/O I/O 192 I/O I/O 156 I/O I/O 193 I/O I/O 157 GND GND 194 I/O I/O 158 I/O I/O 195 I/O I/O 159 I/O I/O 196 I/O I/O 160 I/O I/O 197 I/O I/O 161 I/O I/O 198 I/O I/O 162 I/O I/O 199 I/O I/O 163 I/O I/O 200 I/O I/O 164 VCCI VCCI 201 VCCI VCCI 165 I/O I/O 202 I/O I/O 166 I/O I/O 203 I/O I/O 167 I/O I/O 204 I/O I/O 168 I/O I/O 205 I/O I/O 169 I/O I/O 206 I/O I/O 170 I/O I/O 207 I/O I/O 171 I/O I/O 208 TCK, I/O TCK, I/O 172 I/O I/O 173 I/O I/O 174 I/O I/O 175 I/O I/O 176 I/O I/O 177 I/O I/O 178 I/O QCLKD, I/O 179 I/O I/O 180 CLKA CLKA, I/O 181 CLKB CLKB, I/O 182 NC NC 183 GND GND 184 VCCA VCCA 185 GND GND 3-6 Revision 9 RTSX-SU Radiation-Tolerant FPGAs (UMC) CQ256 1 192 Pin 1 2 191 3 190 4 189 Ceramic Tie Bar 256-Pin CQFP 61 132 62 131 63 130 64 129 Note For Package Manufacturing and Environmental information, visit the Resource Center at http://www.microsemi.com/soc/products/solutions/package/default.aspx. Revision 9 3-7 194 127 65 256 66 255 67 254 68 253 125 196 126 195 193 128 Package Pin Assignments CQ256 CQ256 RTSX32SU RTSX72SU RTSX32SU RTSX72SU Pin Number Function Function Pin Number Function Function 1GND GND 38 I/O I/O 39 I/O I/O 2 TDI, I/O TDI, I/O 3I/O I/O 40 I/O I/O 4I/O I/O 41 I/O I/O 5I/O I/O 42 I/O I/O 6I/O I/O 43 I/O I/O 7I/O I/O 44 I/O I/O 8I/O I/O 45 I/O I/O 9I/O I/O 46 VCCA VCCA 10 I/O I/O 47 I/O VCCI 11 TMS TMS 48 I/O I/O 12 I/O I/O 49 I/O I/O 13 I/O I/O 50 I/O I/O 14 I/O I/O 51 I/O I/O 15 I/O I/O 52 I/O I/O 16 I/O I/O 53 I/O I/O 17 I/O VCCI 54 I/O I/O 18 I/O I/O 55 I/O I/O 19 I/O I/O 56 I/O GND 20 I/O I/O 57 I/O I/O 21 I/O I/O 58 I/O I/O 22 I/O I/O 59 GND GND 23 I/O I/O 60 I/O I/O 24 I/O I/O 61 I/O I/O 25 I/O I/O 62 I/O I/O 26 I/O I/O 63 I/O I/O 27 I/O I/O 64 I/O I/O 28 VCCI VCCI 65 I/O I/O 29 GND GND 66 I/O I/O 30 VCCA VCCA 67 I/O I/O 31 GND GND 68 I/O I/O 32 I/O I/O 69 I/O I/O 33 I/O I/O 70 I/O I/O 34 TRST TRST 71 I/O I/O 35 I/O I/O 72 I/O I/O 36 I/O VCCA 73 I/O VCCI 37 I/O GND 74 I/O I/O 3-8 Revision 9 RTSX-SU Radiation-Tolerant FPGAs (UMC) CQ256 CQ256 RTSX32SU RTSX72SU RTSX32SU RTSX72SU Pin Number Function Function Pin Number Function Function 75 I/O I/O 112 I/O I/O 76 I/O I/O 113 I/O I/O 77 I/O I/O 114 I/O I/O 78 I/O I/O 115 I/O I/O 79 I/O I/O 116 I/O I/O 80 I/O I/O 117 I/O I/O 81 I/O I/O 118 I/O I/O 82 I/O I/O 119 I/O I/O 83 I/O I/O 120 I/O VCCI 84 I/O I/O 121 I/O I/O 85 I/O I/O 122 I/O I/O 86 I/O I/O 123 I/O I/O 87 I/O I/O 124 I/O I/O 88 I/O I/O 125 I/O I/O 89 I/O QCLKA, I/O 126 TDO, I/O TDO, I/O 90 PRB, I/O PRB, I/O 127 I/O I/O 91 GND GND 128 GND GND 92 VCCI VCCI 129 I/O I/O 93 GND GND 130 I/O I/O 94 VCCA VCCA 131 I/O I/O 95 I/O I/O 132 I/O I/O 96 HCLK HCLK 133 I/O I/O 97 I/O I/O 134 I/O I/O 98 I/O QCLKB, I/O 135 I/O I/O 99 I/O I/O 136 I/O I/O 100 I/O I/O 137 I/O I/O 101 I/O I/O 138 I/O I/O 102 I/O I/O 139 I/O I/O 103 I/O I/O 140 I/O I/O 104 I/O I/O 141 VCCA VCCA 105 I/O I/O 142 I/O VCCI 106 I/O I/O 143 I/O GND 107 I/O I/O 144 I/O VCCA 108 I/O I/O 145 I/O I/O 109 I/O I/O 146 I/O I/O 110 GND GND 147 I/O I/O 111 I/O I/O 148 I/O I/O Revision 9 3-9 Package Pin Assignments CQ256 CQ256 RTSX32SU RTSX72SU RTSX32SU RTSX72SU Pin Number Function Function Pin Number Function Function 149 I/O I/O 186 I/O I/O 150 I/O I/O 187 I/O I/O 151 I/O I/O 188 I/O I/O 152 I/O I/O 189 GND GND 153 I/O I/O 190 I/O I/O 154 I/O I/O 191 I/O I/O 155 I/O I/O 192 I/O I/O 156 I/O I/O 193 I/O I/O 157 I/O I/O 194 I/O I/O 158 GND GND 195 I/O I/O 159 NC NC 196 I/O I/O 160 GND GND 197 I/O I/O 161 VCCI VCCI 198 I/O I/O 162 I/O VCCA 199 I/O I/O 163 I/O I/O 200 I/O I/O 164 I/O I/O 201 I/O I/O 165 I/O I/O 202 I/O VCCI 166 I/O I/O 203 I/O I/O 167 I/O I/O 204 I/O I/O 168 I/O I/O 205 I/O I/O 169 I/O I/O 206 I/O I/O 170 I/O I/O 207 I/O I/O 171 I/O I/O 208 I/O I/O 172 I/O I/O 209 I/O I/O 173 I/O I/O 210 I/O I/O 174 VCCA VCCA 211 I/O I/O 175 GND GND 212 I/O I/O 176 GND GND 213 I/O I/O 177 I/O I/O 214 I/O I/O 178 I/O I/O 215 I/O I/O 179 I/O I/O 216 I/O I/O 180 I/O I/O 217 I/O I/O 181 I/O I/O 218 I/O QCLKD, I/O 182 I/O I/O 219 CLKA CLKA, I/O 183 I/O VCCI 220 CLKB CLKB, I/O 184 I/O I/O 221 VCCI VCCI 185 I/O I/O 222 GND GND 3-10 Revision 9 RTSX-SU Radiation-Tolerant FPGAs (UMC) CQ256 RTSX32SU RTSX72SU Pin Number Function Function 223 NC NC 224 GND GND 225 PRA, I/O PRA, I/O 226 I/O I/O 227 I/O I/O 228 I/O VCCA 229 I/O I/O 230 I/O I/O 231 I/O QCLKC, I/O 232 I/O I/O 233 I/O I/O 234 I/O I/O 235 I/O I/O 236 I/O I/O 237 I/O I/O 238 I/O I/O 239 I/O I/O 240 GND GND 241 I/O I/O 242 I/O I/O 243 I/O I/O 244 I/O I/O 245 I/O I/O 246 I/O I/O 247 I/O I/O 248 I/O I/O 249 I/O VCCI 250 I/O I/O 251 I/O I/O 252 I/O I/O 253 I/O I/O 254 I/O I/O 255 I/O I/O 256 TCK, I/O TCK, I/O Revision 9 3-11 Package Pin Assignments CC256 Top View A1 Index Corner 256 193 192 Extenral Wire-Bond Number 1 64 129 65 128 Bottom View T R P N M L K J H G F E D C B A 12 34 56 78 910111213141516 Note For Package Manufacturing and Environmental information, visit the Resource Center at http://www.microsemi.com/soc/products/solutions/package/default.aspx. 3-12 Revision 9 RTSX-SU Radiation-Tolerant FPGAs (UMC) CC256* CC256* External Wire- RTSX32SU External Wire- RTSX32SU Pin Number Bond Number Function Pin Number Bond Number Function A1 1 GND C3 65 GND A2 256 TCK, I/O C4 252 I/O A3 255 I/O C5 249 I/O A4 251 I/O C6 245 I/O A5 243 I/O C7 239 I/O A6 238 I/O C8 230 I/O A7 232 I/O C9 226 CLKA A8 228 I/O C10 218 I/O A9 227 CLKB C11 210 I/O A10 221 I/O C12 201 I/O A11 216 I/O C13 197 I/O A12 209 I/O C14 211 I/O A13 203 I/O C15 178 I/O A14 200 I/O C16 195 I/O A15 2 GND D1 12 I/O A16 13 GND D2 8 I/O B1 242 I/O D3 10 I/O B2 22 GND D4 7 I/O B3 254 I/O D5 250 I/O B4 253 I/O D6 244 I/O B5 248 I/O D7 237 I/O B6 241 I/O D8 229 PRA, I/O B7 234 I/O D9 217 I/O B8 33 VCCA D10 208 I/O B9 222 I/O D11 206 I/O B10 220 I/O D12 199 I/O B11 212 I/O D13 205 I/O B12 207 I/O D14 173 I/O B13 202 I/O D15 190 I/O B14 198 I/O D16 188 I/O B15 32 GND E1 16 I/O B16 196 I/O E2 15 I/O C1 6 I/O E3 9 I/O C2 4 TDI,I/O E4 11 I/O Note: *This table was sorted by the pin number. Note: *This table was sorted by the pin number. Revision 9 3-13 Package Pin Assignments CC256* CC256* External Wire- RTSX32SU External Wire- RTSX32SU Pin Number Bond Number Function Pin Number Bond Number Function E5 5 I/O G7 43 GND E6 240 I/O G8 54 GND E7 233 I/O G9 67 GND E8 231 I/O G10 77 GND E9 223 I/O G11 87 VCCI E10 219 I/O G12 169 I/O E11 213 I/O G13 180 GND E12 167 I/O G14 176 I/O E13 183 I/O G15 179 VCCA E14 189 I/O G16 175 I/O E15 187 I/O H1 29 I/O E16 186 I/O H2 31 I/O F1 17 I/O H3 160 VCCA F2 18 I/O H4 35 TRST F3 20 I/O H5 37 I/O F4 14 TMS H6 108 VCCI F5 19 I/O H7 86 GND F6 28 I/O H8 96 GND F7 3 VCCI H9 107 GND F8 23 VCCI H10 118 GND F9 44 VCCI H11 128 VCCI F10 55 VCCI H12 165 I/O F11 157 I/O H13 170 I/O F12 97 VCCA H14 168 I/O F13 177 I/O H15 166 I/O F14 185 I/O H16 174 I/O F15 184 I/O J1 30 I/O F16 181 I/O J2 38 I/O G1 24 I/O J3 40 I/O G2 25 I/O J4 41 I/O G3 27 I/O J5 39 I/O G4 26 I/O J6 139 VCCI G5 21 I/O J7 127 GND G6 66 VCCI J8 140 GND Note: *This table was sorted by the pin number. Note: *This table was sorted by the pin number. 3-14 Revision 9 RTSX-SU Radiation-Tolerant FPGAs (UMC) CC256* CC256* External Wire- RTSX32SU External Wire- RTSX32SU Pin Number Bond Number Function Pin Number Bond Number Function J9 151 GND L11 103 I/O J10 161 GND L12 149 I/O J11 150 VCCI L13 146 I/O J12 159 I/O L14 148 I/O J13 163 I/O L15 145 I/O J14 164 I/O L16 147 I/O J15 162 I/O M1 42 I/O J16 158 I/O M2 53 I/O K1 34 I/O M3 61 I/O K2 45 I/O M4 60 I/O K3 47 I/O M5 72 I/O K4 50 VCCA M6 81 I/O K5 48 I/O M7 89 I/O K6 171 VCCI M8 95 PRB, I/O K7 172 GND M9 101 I/O K8 182 GND M10 105 I/O K9 192 GND M11 114 I/O K10 204 GND M12 111 I/O K11 191 VCCI M13 141 I/O K12 153 I/O M14 142 I/O K13 155 I/O M15 137 I/O K14 156 I/O M16 144 I/O K15 152 I/O N1 49 I/O K16 154 I/O N2 57 I/O L1 36 I/O N3 63 I/O L2 46 I/O N4 79 I/O L3 51 I/O N5 70 I/O L4 58 I/O N6 76 I/O L5 52 I/O N7 83 I/O L6 91 I/O N8 99 I/O L7 194 VCCI N9 109 I/O L8 214 VCCI N10 117 I/O L9 235 VCCI N11 112 I/O L10 246 VCCI N12 124 I/O Note: *This table was sorted by the pin number. Note: *This table was sorted by the pin number. Revision 9 3-15 Package Pin Assignments CC256* CC256* External Wire- RTSX32SU External Wire- RTSX32SU Pin Number Bond Number Function Pin Number Bond Number Function N13 121 I/O R15 225 GND N14 133 I/O R16 193 GND N15 135 I/O T1 236 GND N16 136 I/O T2 69 I/O P1 59 I/O T3 71 I/O P2 138 GND T4 75 I/O P3 56 I/O T5 80 I/O P4 74 I/O T6 84 I/O P5 64 I/O T7 88 I/O P6 82 I/O T8 93 I/O P7 90 I/O T9 224 VCCA P8 94 I/O T10 102 I/O P9 104 I/O T11 110 I/O P10 113 I/O T12 116 I/O P11 119 I/O T13 122 I/O P12 123 I/O T14 125 I/O P13 143 VCCA T15 129 TDO,I/O P14 131 I/O T16 247 GND P15 132 I/O Note: *This table was sorted by the pin number. P16 134 I/O R1 62 I/O R2 215 GND R3 68 I/O R4 73 I/O R5 78 I/O R6 85 I/O R7 92 I/O R8 98 I/O R9 100 HCLK R10 106 I/O R11 115 I/O R12 120 I/O R13 126 I/O R14 130 I/O Note: *This table was sorted by the pin number. 3-16 Revision 9 RTSX-SU Radiation-Tolerant FPGAs (UMC) CC256* CC256* External Wire- RTSX32SU External Wire- RTSX32SU Pin Number Bond Number Function Pin Number Bond Number Function A1 1 GND H4 35 TRST A15 2 GND L1 36 I/O F7 3 VCCI H5 37 I/O C2 4 TDI,I/O J2 38 I/O E5 5 I/O J5 39 I/O C1 6 I/O J3 40 I/O D4 7 I/O J4 41 I/O D2 8 I/O M1 42 I/O E3 9 I/O G7 43 GND D3 10 I/O F9 44 VCCI E4 11 I/O K2 45 I/O D1 12 I/O L2 46 I/O A16 13 GND K3 47 I/O F4 14 TMS K5 48 I/O E2 15 I/O N1 49 I/O E1 16 I/O K4 50 VCCA F1 17 I/O L3 51 I/O F2 18 I/O L5 52 I/O F5 19 I/O M2 53 I/O F3 20 I/O G8 54 GND G5 21 I/O F10 55 VCCI B2 22 GND P3 56 I/O F8 23 VCCI N2 57 I/O G1 24 I/O L4 58 I/O G2 25 I/O P1 59 I/O G4 26 I/O M4 60 I/O G3 27 I/O M3 61 I/O F6 28 I/O R1 62 I/O H1 29 I/O N3 63 I/O J1 30 I/O P5 64 I/O H2 31 I/O C3 65 GND B15 32 GND G6 66 VCCI B8 33 VCCA G9 67 GND K1 34 I/O R3 68 I/O Note: *This table was sorted by the wire-bond number. Note: *This table was sorted by the wire-bond number. Revision 9 3-17 Package Pin Assignments CC256* CC256* External Wire- RTSX32SU External Wire- RTSX32SU Pin Number Bond Number Function Pin Number Bond Number Function T2 69 I/O L11 103 I/O N5 70 I/O P9 104 I/O T3 71 I/O M10 105 I/O M5 72 I/O R10 106 I/O R4 73 I/O H9 107 GND P4 74 I/O H6 108 VCCI T4 75 I/O N9 109 I/O N6 76 I/O T11 110 I/O G10 77 GND M12 111 I/O R5 78 I/O N11 112 I/O N4 79 I/O P10 113 I/O T5 80 I/O M11 114 I/O M6 81 I/O R11 115 I/O P6 82 I/O T12 116 I/O N7 83 I/O N10 117 I/O T6 84 I/O H10 118 GND R6 85 I/O P11 119 I/O H7 86 GND R12 120 I/O G11 87 VCCI N13 121 I/O T7 88 I/O T13 122 I/O M7 89 I/O P12 123 I/O P7 90 I/O N12 124 I/O L6 91 I/O T14 125 I/O R7 92 I/O R13 126 I/O T8 93 I/O J7 127 GND P8 94 I/O H11 128 VCCI M8 95 PRB, I/O T15 129 TDO,I/O H8 96 GND R14 130 I/O F12 97 VCCA P14 131 I/O R8 98 I/O P15 132 I/O N8 99 I/O N14 133 I/O R9 100 HCLK P16 134 I/O M9 101 I/O N15 135 I/O T10 102 I/O N16 136 I/O Note: *This table was sorted by the wire-bond number. Note: *This table was sorted by the wire-bond number. 3-18 Revision 9 RTSX-SU Radiation-Tolerant FPGAs (UMC) CC256* CC256* External Wire- RTSX32SU External Wire- RTSX32SU Pin Number Bond Number Function Pin Number Bond Number Function M15 137 I/O K6 171 VCCI P2 138 GND K7 172 GND J6 139 VCCI D14 173 I/O J8 140 GND H16 174 I/O M13 141 I/O G16 175 I/O M14 142 I/O G14 176 I/O P13 143 VCCA F13 177 I/O M16 144 I/O C15 178 I/O L15 145 I/O G15 179 VCCA L13 146 I/O G13 180 GND L16 147 I/O F16 181 I/O L14 148 I/O K8 182 GND L12 149 I/O E13 183 I/O J11 150 VCCI F15 184 I/O J9 151 GND F14 185 I/O K15 152 I/O E16 186 I/O K12 153 I/O E15 187 I/O K16 154 I/O D16 188 I/O K13 155 I/O E14 189 I/O K14 156 I/O D15 190 I/O F11 157 I/O K11 191 VCCI J16 158 I/O K9 192 GND J12 159 I/O R16 193 GND H3 160 VCCA L7 194 VCCI J10 161 GND C16 195 I/O J15 162 I/O B16 196 I/O J13 163 I/O C13 197 I/O J14 164 I/O B14 198 I/O H12 165 I/O D12 199 I/O H15 166 I/O A14 200 I/O E12 167 I/O C12 201 I/O H14 168 I/O B13 202 I/O G12 169 I/O A13 203 I/O H13 170 I/O K10 204 GND Note: *This table was sorted by the wire-bond number. Note: *This table was sorted by the wire-bond number. Revision 9 3-19 Package Pin Assignments CC256* CC256* External Wire- RTSX32SU External Wire- RTSX32SU Pin Number Bond Number Function Pin Number Bond Number Function D13 205 I/O C7 239 I/O D11 206 I/O E6 240 I/O B12 207 I/O B6 241 I/O D10 208 I/O B1 242 I/O A12 209 I/O A5 243 I/O C11 210 I/O D6 244 I/O C14 211 I/O C6 245 I/O B11 212 I/O L10 246 VCCI E11 213 I/O T16 247 GND L8 214 VCCI B5 248 I/O R2 215 GND C5 249 I/O A11 216 I/O D5 250 I/O D9 217 I/O A4 251 I/O C10 218 I/O C4 252 I/O E10 219 I/O B4 253 I/O B10 220 I/O B3 254 I/O A10 221 I/O A3 255 I/O B9 222 I/O A2 256 TCK, I/O E9 223 I/O Note: *This table was sorted by the wire-bond number. T9 224 VCCA R15 225 GND C9 226 CLKA A9 227 CLKB A8 228 I/O D8 229 PRA, I/O C8 230 I/O E8 231 I/O A7 232 I/O E7 233 I/O B7 234 I/O L9 235 VCCI T1 236 GND D7 237 I/O A6 238 I/O Note: *This table was sorted by the wire-bond number. 3-20 Revision 9 RTSX-SU Radiation-Tolerant FPGAs (UMC) CG624 25 24 23 22 21 20 19 18 17 16 15 14 13 12 11 10 9 8 7 6 5 4 3 2 1 A B C D E F G H J K L M N P R T U V W Y AA AB AC AD AE Note For Package Manufacturing and Environmental information, visit the Resource Center at http://www.microsemi.com/soc/products/solutions/package/default.aspx. Revision 9 3-21 Package Pin Assignments CG624 CG624 CG624 RTSX72SU RTSX72SU RTSX72SU Pin Number Function Pin Number Function Pin Number Function A2 NC B12 I/O C22 I/O A3 NC B13 I/O C23 GND A4 NC B14 CLKB, I/O C24 VCCI A5 I/O B15 I/O C25 NC A6 I/O B16 I/O D1 GND A7 I/O B17 I/O D2 GND A8 I/O B18 I/O D3 TDI, I/O A9 I/O B19 I/O D4 GND A10 I/O B20 I/O D5 I/O A11 I/O B21 I/O D6 I/O A12 I/O B22 GND D7 I/O A13 GND B23 VCCI D8 I/O A14 I/O B24 GND D9 I/O A15 I/O B25 NC D10 I/O A16 I/O C1 NC D11 I/O A17 I/O C2 VCCI D12 I/O A18 I/O C3 GND D13 I/O A19 I/O C4 I/O D14 QCLKD, I/O A20 I/O C5 I/O D15 I/O A21 I/O C6 I/O D16 I/O A22 GND C7 I/O D17 I/O A23 NC C8 I/O D18 I/O A24 NC C9 I/O D19 I/O A25 NC C10 I/O D20 I/O B1 NC C11 QCLKC, I/O D21 I/O B2 GND C12 I/O D22 VCCI B3 GND C13 PRA, I/O D23 GND B4 VCCI C14 CLKA, I/O D24 GND B5 GND C15 I/O D25 GND B6 I/O C16 I/O E1 I/O B7 I/O C17 I/O E2 I/O B8 VCCI C18 I/O E3 I/O B9 GND C19 I/O E4 I/O B10 I/O C20 I/O E5 TCK, I/O B11 I/O C21 I/O E6 I/O 3-22 Revision 9 RTSX-SU Radiation-Tolerant FPGAs (UMC) CG624 CG624 CG624 RTSX72SU RTSX72SU RTSX72SU Pin Number Function Pin Number Function Pin Number Function E7 I/O F17 I/O H2 I/O E8 I/O F18 I/O H3 I/O E9 I/O F19 I/O H4 I/O E10 I/O F20 I/O H5 I/O E11 I/O F21 I/O H6 I/O E12 VCCA F22 I/O H7 I/O E13 GND F23 I/O H8 VCCI E14 I/O F24 I/O H9 NC E15 I/O F25 I/O H10 NC E16 I/O G1 I/O H11 NC E17 I/O G2 I/O H12 NC E18 I/O G3 TMS H13 NC E19 I/O G4 I/O H14 NC E20 I/O G5 I/O H15 NC E21 I/O G6 I/O H16 NC E22 I/O G7 VCCI H17 NC E23 I/O G8 NC H18 VCCI E24 I/O G9 NC H19 I/O E25 I/O G10 NC H20 I/O F1 I/O G11 NC H21 I/O F2 VCCI G12 NC H22 I/O F3 I/O G13 NC H23 I/O F4 I/O G14 NC H24 GND F5 I/O G15 NC H25 I/O F6 NC G16 NC J1 I/O F7 NC G17 NC J2 I/O F8 I/O G18 GND J3 I/O F9 NC G19 VCCI J4 I/O F10 NC G20 I/O J5 I/O F11 NC G21 I/O J6 I/O F12 NC G22 I/O J7 NC F13 I/O G23 I/O J8 NC F14 I/O G24 I/O J9 VCCI F15 NC G25 I/O J10 NC F16 GND H1 I/O J11 NC Revision 9 3-23 Package Pin Assignments CG624 CG624 CG624 RTSX72SU RTSX72SU RTSX72SU Pin Number Function Pin Number Function Pin Number Function J12 NC K22 I/O M7 NC J13 NC K23 I/O M8 NC J14 NC K24 I/O M9 NC J15 NC K25 I/O M10 GND J16 NC L1 I/O M11 GND J17 VCCI L2 I/O M12 GND J18 NC L3 I/O M13 GND J19 NC L4 I/O M14 GND J20 I/O L5 I/O M15 GND J21 VCCA L6 I/O M16 GND J22 I/O L7 NC M17 NC J23 I/O L8 NC M18 NC J24 I/O L9 NC M19 NC J25 I/O L10 GND M20 I/O K1 I/O L11 GND M21 GND K2 GND L12 GND M22 I/O K3 I/O L13 GND M23 I/O K4 I/O L14 GND M24 GND K5 I/O L15 GND M25 I/O K6 GND L16 GND N1 I/O K7 NC L17 NC N2 I/O K8 NC L18 NC N3 I/O K9 NC L19 NC N4 I/O K10 GND L20 I/O N5 VCCA K11 GND L21 I/O N6 I/O K12 GND L22 I/O N7 VCCA K13 GND L23 I/O N8 NC K14 GND L24 I/O N9 NC K15 GND L25 I/O N10 GND K16 GND M1 I/O N11 GND K17 NC M2 I/O N12 GND K18 NC M3 I/O N13 GND K19 NC M4 I/O N14 GND K20 I/O M5 GND N15 GND K21 I/O M6 I/O N16 GND 3-24 Revision 9 RTSX-SU Radiation-Tolerant FPGAs (UMC) CG624 CG624 CG624 RTSX72SU RTSX72SU RTSX72SU Pin Number Function Pin Number Function Pin Number Function N17 NC R2 I/O T12 GND N18 NC R3 I/O T13 GND N19 VCCA R4 TRST T14 GND N20 I/O R5 I/O T15 GND N21 VCCA R6 GND T16 GND N22 I/O R7 NC T17 NC N23 I/O R8 NC T18 NC N24 VCCI R9 NC T19 NC N25 I/O R10 GND T20 GND P1 I/O R11 GND T21 I/O P2 I/O R12 GND T22 I/O P3 I/O R13 GND T23 I/O P4 I/O R14 GND T24 I/O P5 I/O R15 GND T25 I/O P6 I/O R16 GND U1 I/O P7 NC R17 NC U2 I/O P8 NC R18 NC U3 I/O P9 NC R19 NC U4 I/O P10 GND R20 I/O U5 I/O P11 GND R21 I/O U6 I/O P12 GND R22 I/O U7 I/O P13 GND R23 I/O U8 NC P14 GND R24 I/O U9 VCCI P15 GND R25 I/O U10 NC P16 GND T1 I/O U11 NC P17 NC T2 I/O U12 NC P18 NC T3 I/O U13 NC P19 NC T4 I/O U14 NC P20 I/O T5 I/O U15 NC P21 GND T6 I/O U16 NC P22 I/O T7 I/O U17 VCCI P23 I/O T8 NC U18 NC P24 I/O T9 NC U19 NC P25 I/O T10 GND U20 I/O R1 I/O T11 GND U21 I/O Revision 9 3-25 Package Pin Assignments CG624 CG624 CG624 RTSX72SU RTSX72SU RTSX72SU Pin Number Function Pin Number Function Pin Number Function U22 I/O W7 VCCI Y17 I/O U23 I/O W8 NC Y18 I/O U24 I/O W9 NC Y19 I/O U25 I/O W10 NC Y20 I/O V1 I/O W11 NC Y21 I/O V2 I/O W12 NC Y22 I/O V3 I/O W13 NC Y23 I/O V4 VCCA W14 NC Y24 GND V5 I/O W15 NC Y25 I/O V6 I/O W16 NC AA1 GND V7 GND W17 NC AA2 GND V8 VCCI W18 I/O AA3 I/O V9 NC W19 VCCI AA4 I/O V10 NC W20 I/O AA5 GND V11 NC W21 I/O AA6 I/O V12 NC W22 I/O AA7 I/O V13 NC W23 I/O AA8 I/O V14 NC W24 I/O AA9 I/O V15 NC W25 I/O AA10 I/O V16 NC Y1 I/O AA11 I/O V17 NC Y2 I/O AA12 I/O V18 VCCI Y3 I/O AA13 VCCA V19 I/O Y4 I/O AA14 GND V20 I/O Y5 I/O AA15 I/O V21 I/O Y6 I/O AA16 I/O V22 VCCA Y7 I/O AA17 I/O V23 I/O Y8 I/O AA18 I/O V24 I/O Y9 I/O AA19 I/O V25 I/O Y10 I/O AA20 I/O W1 I/O Y11 NC AA21 GND W2 VCCI Y12 GND AA22 I/O W3 I/O Y13 I/O AA23 I/O W4 I/O Y14 NC AA24 I/O W5 I/O Y15 GND AA25 GND W6 I/O Y16 I/O AB1 NC 3-26 Revision 9 RTSX-SU Radiation-Tolerant FPGAs (UMC) CG624 CG624 CG624 RTSX72SU RTSX72SU RTSX72SU Pin Number Function Pin Number Function Pin Number Function AB2 VCCI AC12 PRB, I/O AD22 GND AB3 I/O AC13 I/O AD23 VCCI AB4 GND AC14 HCLK AD24 GND AB5 I/O AC15 I/O AD25 NC AB6 I/O AC16 I/O AE1 NC AB7 I/O AC17 I/O AE2 NC AB8 I/O AC18 I/O AE3 NC AB9 I/O AC19 I/O AE4 GND AB10 I/O AC20 I/O AE5 I/O AB11 I/O AC21 I/O AE6 I/O AB12 QCLKA, I/O AC22 I/O AE7 I/O AB13 I/O AC23 GND AE8 I/O AB14 I/O AC24 I/O AE9 I/O AB15 I/O AC25 NC AE10 I/O AB16 I/O AD1 NC AE11 I/O AB17 I/O AD2 GND AE12 I/O AB18 I/O AD3 VCCI AE13 I/O AB19 I/O AD4 GND AE14 QCLKB, I/O AB20 I/O AD5 I/O AE15 I/O AB21 TDO, I/O AD6 I/O AE16 I/O AB22 VCCI AD7 I/O AE17 I/O AB23 I/O AD8 I/O AE18 I/O AB24 VCCI AD9 I/O AE19 I/O AB25 NC AD10 VCCI AE20 I/O AC1 NC AD11 I/O AE21 I/O AC2 I/O AD12 I/O AE22 GND AC3 GND AD13 I/O AE23 NC AC4 I/O AD14 I/O AE24 NC AC5 I/O AD15 I/O AE25 NC AC6 I/O AD16 GND AC7 I/O AD17 I/O AC8 I/O AD18 I/O AC9 I/O AD19 I/O AC10 I/O AD20 I/O AC11 I/O AD21 I/O Revision 9 3-27 4 – Datasheet Information List of Changes The following table lists critical changes that were made in each version of the document. Revision Changes Page Revision 9 Notes were added to Table 3 • Extended Flow for RTSX32SU and RTSX72SU1,2 and v, vi (March 2012) Table 4 • EV Flow (Class V Equivalent Flow Processing) for RTSX32SU and RTSX72SU1, 2, 3 stating that X-Ray inspection is not performed on RTSX72SU in CQ208 and CQ256 packages because these packages contain a heat-spreader that prevents the X-Rays from penetrating through (SAR 37260). Revision 8 The "Designed for Space" section bullet regarding single event latch-up immunity was i (January 2012) revised to "Single Event Latch-Up (SEL) Immunity to LET > 104 MeVcm2/mg" (SAR TH 33213). The "Features" section and "Security Fuses" section were revised to clarify that i, 2-47 although no existing security measures can give an absolute guarantee, Microsemi FPGAs implement the best security available in the industry (SAR 34672). Package names used throughout the document the were revised to match standards i, 3-1 given in Package Mechanical Drawings (SAR 34783). Notes stating that All CCGA PROTOs will be offered in Six Sigma Columns were ii, iii removed from the "Ordering Information" section and "Temperature Grade and Application Offering" section (SAR 36171). A note was added to the "EV Flow (Class V Flow Equivalent Processing)" table vi regarding an enhancement to the Au ball bonding process control (SAR 36172). References to Silicon Sculptor II were changed to Silicon Sculptor series programmers 1-6, (SAR 36473). 2-47 The following note was added to Table 2-3 • Absolute Maximum Conditions1 (SAR 2-2 32303): For AC signals, the 5 V non-PCI input signals may overshoot during transitions to VCCI + 1.2 V for no longer than 11 ns. The 3.3V non-PCI input signals may overshoot during transitions to 6.0 V for no longer than 11 ns. Current during the transition must not exceed 95 mA. Refer to PCI specifications for the PCI input overshoot limit. EQ 2 was changed from PDC = (ICC) * VCCA + (ICC) * VCCI to PDC = ICCA * VCCA + ICCI * VCCI (SAR 35679). The "Hot Swapping" section was revised. Table 2-3 • Time at which I/Os Become Active 2-13, by Ramp Rate was deleted. The information from this table is in the Microsemi SX-A 2-1 and RTSX-SU Devices in Hot-Swap and Cold-Sparing Applications application note (SAR 35624). The equation for slew rate, which first appears in the note for Table 2-13 • RTSX32SU 2-18 5 V TTL and 3.3 V LVTTL I/O Module, was revised by adding "±." It is negative for falling edges and positive for rising edges. The equation was revised in each place it occurs in the datasheet (SAR 30955). Revision 7 The range for VCCA DC core supply voltage in Table2-3 • Absolute Maximum 2-2 (October 2010) Conditions1 was erroneously changed in Revision 6 of the datasheet from "–0.3 to 3.0" to "0.3 to 3.0." It has now been changed back to the correct value of "–0.3 to 3.0." Revision 9 4-1 Datasheet Information Revision Changes Page Revision 6 The versioning system for datasheets has been changed. Datasheets are assigned a N/A (May 2010) revision number that increments each time the datasheet is revised. The "RTSX-SU Device Status" table indicates the status for each device in the family. The "Processing Flows" section and "Prototyping Options" section are new. i The "Ordering Information" table was updated to add the EV and PROTO application ii (temperature range) ordering codes. A note was added to the "Ordering Information" table and "Temperature Grade and ii Application Offering" table to explain that PROTO refers to RTSX-SU prototype units. All CCGA PROTOs will be offered in Six Sigma Columns. The note for the "Ceramic Device Resources" table regarding availability of the 256-pin iii CCLG package for military temperature only was deleted. EV and PROTO were added to the "Temperature Grade and Application Offering" table. iii The B and E codes were also added for the CG256 package for RTSX32SU. The "Speed Grade and Temperature Grade Matrix" table was replaced. iii A note regarding performing CCGA tests at LGA level was added to Table 2 • MIL-STD- iv, v 883 Class B Product Flow* for RTSX32SU and RTSX72SU and Table 3 • Extended Flow for RTSX32SU and RTSX72SU1,2. Table 4 • EV Flow (Class V Equivalent Flow Processing) for RTSX32SU and vi RTSX72SU1, 2, 3 is new. The "Low-Cost Prototyping Solution" section was expanded to include prototyping with 1-7 RTSX-SU PROTO units. A note was added to Table 2-2 • Characteristics for All I/O Configurations, giving 2-1 information about power-up resistor pull. Table 2-3 • Absolute Maximum Conditions1 was revised. The TJ and VO parameters 2-2 and some notes were added. Temperature range and the ICC standby current parameter were added to Table 2-4 • 2-2 Recommended Operating Conditions (SAR 62430). Table 2-5 • RTSX-SU Standby Current is new. 2-3 The "AC Power Dissipation" section was revised, including the addition of two formulas 2-4 for power dissipation for the RTSX72SU device. Additional parameters were added to Table 2-6 • Fixed Power Parameters. The definition of C given in relation to EQ 6 was L changed to "output load capacitance in pF" (SAR 69980). A note was added to the "User I/O" section to exercise care when using JTAG to tristate 2-13 all I/Os (SAR 79515). The "Using the Weak Pull-Up and Pull-Down Circuits" section is new (SAR 79515). 2-14 The minimum value for VIL (– 0.5 V) was added to Table 2-12 • 5 V TTL and 3.3 V 2-16, LVTTL Electrical Specifications and Table 2-15 • 5 V CMOS Electrical Specifications 2-19 (SAR 41193). The second table note, regarding overshoot for AC signals, was deleted (SAR 68437). Values in the following tables were revised (SAR 68197). The t parameter was 2-17, ENHZS added where applicable. 2-18, 2-20, Table 2-13 • RTSX32SU 5 V TTL and 3.3 V LVTTL I/O Module 2-21 Table 2-14 • RTSX72SU 5 V TTL and 3.3 V LVTTL I/O Module, Table 2-16 • RTSX32SU 5 V CMOS I/O Module Table 2-17 • RTSX72SU 5 V CMOS I/O Module 4-2 Revision 9 RTSX-SU Radiation-Tolerant FPGAs (UMC) Revision Changes Page Revision 6 A note regarding undershoot for AC signals was added to Table 2-18 • 5 V PCI DC 2-22 (continued) Specifications (SAR 68437). Values in the following tables were revised (SAR 68197). 2-24 Table 2-20 • RTSX32SU 5 V PCI I/O Module Table 2-21 • RTSX72SU 5 V PCI I/O Module Notes regarding undershoot and overshoot for AC signals were added to Table 2-22 • 2-26 3.3 V PCI DC Specifications (SAR 68437). Values in the following tables were revised (SAR 68197). 2-28 Table 2-24 • RTSX32SU 3.3 V PCI I/O Module Table 2-25 • RTSX72SU 3.3 V PCI I/O Module The note in the "CQ208" table stating that pin 65 is a no connect on commercial 3-4 A54SX32S PQ208 was deleted (SAR 52216). v2.2 The notes in Table 2-12 • 5 V TTL and 3.3 V LVTTL Electrical Specifications were 2-16 (March 2006) updated. The notes in Table 2-15 • 5 V CMOS Electrical Specifications were updated. 2-19 v2.1 Figure 1-5 • Probe Setup was updated and a note was added. 1-8 Table 2-12 • 5 V TTL and 3.3 V LVTTL Electrical Specifications was updated to include 2-16 Notes 2 and 3. Table 2-15 • 5 V CMOS Electrical Specifications was updated to include Notes 1 and 2. 2-19 Footnote 1 and Footnote 2 in the "Pin Descriptions" section were updated. 2-11, 2-12 v2.0 The "RTSX-SU Product Profile" table was updated to include the CQ84. i The "Ceramic Device Resources" table was updated to include CQ84. iii The "Temperature Grade and Application Offering" table was updated to include CQ84. iii Table 2-3 • Time at which I/Os Become Active by Ramp Rate was updated. The 2-1 0.25V/ms was changed to 0.25V/μs and 0.025V/ms was changed to 0.025V/µs. Table 2-7 • Package Thermal Characteristics was updated to include the CQ84. 2-8 Table 2-13 • RTSX32SU 5 V TTL and 3.3 V LVTTL I/O Module through Table 2-17 • 2-17 to RTSX72SU 5 V CMOS I/O Module, Table 2-20 • RTSX32SU 5 V PCI I/O Module, 2-24, Table 2-21 • RTSX72SU 5 V PCI I/O Module, Table 2-24 • RTSX32SU 3.3 V PCI I/O 2-28 Module, and Table 2-25 • RTSX72SU 3.3 V PCI I/O Module were updated to include Note 2. The headings in Table2-34 • Boundary Scan Pin Functionality were updated to 2-8 Dedicated Test Mode and Flexible Mode. The "CQ84" section, which includes the package figure and the pin table, is new. 3-1 Advance v0.3 In Table 2-15 • 5 V CMOS Electrical Specifications, the I = –20μA and I = ±20 µA. 2-19 OH OL Revision 9 4-3 Datasheet Information Revision Changes Page Advance v0.2 Table 2-8 • Temperature and Voltage Derating Factors was updated. 2-9 The following tables were updated: 2-17 through Table 2-13 • RTSX32SU 5 V TTL and 3.3 V LVTTL I/O Module 2-42 Table 2-14 • RTSX72SU 5 V TTL and 3.3 V LVTTL I/O Module Table 2-16 • RTSX32SU 5 V CMOS I/O Module, Table 2-17 • RTSX72SU 5 V CMOS I/O Module Table 2-20 • RTSX32SU 5 V PCI I/O Module Table 2-21 • RTSX72SU 5 V PCI I/O Module Table 2-24 • RTSX32SU 3.3 V PCI I/O Module Table 2-25 • RTSX72SU 3.3 V PCI I/O Module Table 2-27 • R-Cell through Table 2-33 • RTSX72SU at VCCI = 4.5 V 4-4 Revision 9 RTSX-SU Radiation-Tolerant FPGAs (UMC) Datasheet Categories In order to provide the latest information to designers, some datasheets are published before data has been fully characterized from silicon devices. The data provided for a given device, as highlighted in the "RTSX-SU Device Status" table, is designated as "Product Brief," "Advance," "Production," or "Datasheet Supplement." The definitions of these categories are as follows: Product Brief The product brief is a summarized version of a datasheet (advanced or production) containing general product information. This brief gives an overview of specific device and family information. Advance This datasheet version contains initial estimated information based on simulation, other products, devices, or speed grades. This information can be used as estimates, but not for production. Unmarked (production) This datasheet version contains information that is considered to be final. Datasheet Supplement The datasheet supplement gives specific device information for a derivative family that differs from the general family datasheet. The supplement is to be used in conjunction with the datasheet to obtain more detailed information and for specifications that do not differ between the two families. Export Administration Regulations (EAR) or International Traffic in Arms Regulations (ITAR) The product described in this datasheet could be subject to either the Export Administration Regulations (EAR) or in some cases the International Traffic in Arms Regulations (ITAR). They could require an approved export license prior to export from the United States. An export includes release of product or disclosure of technology to a foreign national inside or outside the United States. Revision 9 4-5 Microsemi Corporation (NASDAQ: MSCC) offers a comprehensive portfolio of semiconductor solutions for: aerospace, defense and security; enterprise and communications; and industrial and alternative energy markets. Products include high-performance, high-reliability analog and RF devices, mixed signal and RF integrated circuits, customizable SoCs, FPGAs, and complete subsystems. Microsemi is headquartered in Aliso Viejo, Calif. Learn more at www.microsemi.com. Microsemi Corporate Headquarters One Enterprise Drive, Aliso Viejo CA 92656 © 2012 Microsemi Corporation. All rights reserved. Microsemi and the Microsemi logo are trademarks of Within the USA: (800) 713-4113 Microsemi Corporation. All other trademarks and service marks are the property of their respective owners. Outside the USA: (949) 221-7100 Fax: (949) 756-0308 · www.microsemi.com 51700053-9/3.12
Frequently asked questions
What makes Elite.Parts unique?

What kind of warranty will the RTSX72SU have?

Which carriers does Elite.Parts work with?

Will Elite.Parts sell to me even though I live outside the USA?

I have a preferred payment method. Will Elite.Parts accept it?

Why buy from GID?
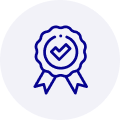
Quality
We are industry veterans who take pride in our work
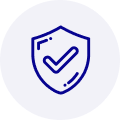
Protection
Avoid the dangers of risky trading in the gray market
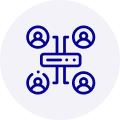
Access
Our network of suppliers is ready and at your disposal
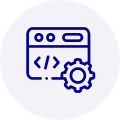
Savings
Maintain legacy systems to prevent costly downtime
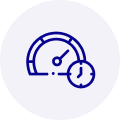
Speed
Time is of the essence, and we are respectful of yours
What they say about us
FANTASTIC RESOURCE
One of our top priorities is maintaining our business with precision, and we are constantly looking for affiliates that can help us achieve our goal. With the aid of GID Industrial, our obsolete product management has never been more efficient. They have been a great resource to our company, and have quickly become a go-to supplier on our list!
Bucher Emhart Glass
EXCELLENT SERVICE
With our strict fundamentals and high expectations, we were surprised when we came across GID Industrial and their competitive pricing. When we approached them with our issue, they were incredibly confident in being able to provide us with a seamless solution at the best price for us. GID Industrial quickly understood our needs and provided us with excellent service, as well as fully tested product to ensure what we received would be the right fit for our company.
Fuji
HARD TO FIND A BETTER PROVIDER
Our company provides services to aid in the manufacture of technological products, such as semiconductors and flat panel displays, and often searching for distributors of obsolete product we require can waste time and money. Finding GID Industrial proved to be a great asset to our company, with cost effective solutions and superior knowledge on all of their materials, it’d be hard to find a better provider of obsolete or hard to find products.
Applied Materials
CONSISTENTLY DELIVERS QUALITY SOLUTIONS
Over the years, the equipment used in our company becomes discontinued, but they’re still of great use to us and our customers. Once these products are no longer available through the manufacturer, finding a reliable, quick supplier is a necessity, and luckily for us, GID Industrial has provided the most trustworthy, quality solutions to our obsolete component needs.
Nidec Vamco
TERRIFIC RESOURCE
This company has been a terrific help to us (I work for Trican Well Service) in sourcing the Micron Ram Memory we needed for our Siemens computers. Great service! And great pricing! I know when the product is shipping and when it will arrive, all the way through the ordering process.
Trican Well Service
GO TO SOURCE
When I can't find an obsolete part, I first call GID and they'll come up with my parts every time. Great customer service and follow up as well. Scott emails me from time to time to touch base and see if we're having trouble finding something.....which is often with our 25 yr old equipment.
ConAgra Foods